Chapter 5. Sensation and Perception
The Other Senses
Jessica Motherwell McFarlane
Approximate reading time: 39 minutes
Vision and hearing have received an incredible amount of attention from researchers over the years. While there is still much to be learned about how these sensory systems work, we have a much better understanding of them than of our other important sensory modalities. In this section, we will explore our chemical senses (taste and smell) and our body senses of touch (proprioception), temperature (thermoception), pain (nociception), balance (equilibrioception), body position (vestibular sense), and inner body sensations (interoception).
Taste and Smell: Chemical-based senses
Taste (gustation) and smell (olfaction) are called chemical senses because both have sensory receptors that respond to molecules in the food we eat or in the air we breathe. There is a pronounced interaction between our chemical senses. For example, when we describe the flavour of a given food, we are really referring to both gustatory and olfactory properties of the food working in combination.
Taste (Gustation)
You may have learned since elementary school that there are four basic groupings of taste: sweet, salty, sour, and bitter. Research demonstrates, however, that we have at least six taste groupings. Umami is our fifth taste. Umami is a Japanese word that roughly translates to yummy, and it is associated with a taste for monosodium glutamate (Kinnamon & Vandenbeuch, 2009). There is also a growing body of experimental evidence suggesting that we possess a taste for the fatty content of a given food (Mizushige, Inoue, & Fushiki, 2007). The term for this sixth taste is oleogustus.
Monosodium Glutamate (MSG)
Monosodium glutamate (MSG) is a flavour enhancer that imparts a savoury taste to food, often described as umami, which is considered one of the six basic tastes alongside sweet, sour, bitter, salty and oleogustus. MSG is found naturally in some foods and is also added to others to enhance their flavour. Here are some examples of foods that contain MSG:
Naturally occurring MSG
- Tomatoes and tomato products like tomato sauce and tomato paste.
- Parmesan and other aged cheeses.
- Seaweed, particularly kombu, used in making dashi (a Japanese broth).
- Soy sauce and fermented soy products like miso and tempeh.
- Mushrooms, especially shiitake mushrooms.
Processed foods with added MSG
- Packaged snacks such as chips and flavoured crackers.
- Instant noodle products and soup mixes.
- Frozen or canned meals, including frozen pizzas and TV dinners.
- Processed meats, including sausages, hot dogs, and canned meats.
- Seasoning blends and stock cubes.
MSG enhances the umami flavour, making foods taste more savoury and satisfying.
While it’s a subject of controversy regarding health effects, it is generally recognised as safe by food safety authorities when consumed in typical amounts found in foods.
Molecules from the food and beverages we consume dissolve in our saliva and interact with taste receptors on our tongue and in our mouth and throat. Taste buds are formed by groupings of taste receptor cells with hair-like extensions that protrude into the central pore of the taste bud (Figure SP.14). Taste buds have a life cycle of ten days to two weeks, so even destroying some by burning your tongue won’t have any long-term effect; they just grow right back. Taste molecules bind to receptors on this extension and cause chemical changes within the sensory cell that result in neural impulses being transmitted to the brain via different nerves, depending on where the receptor is located. Taste information is transmitted to the medulla, thalamus, and limbic system, and to the gustatory cortex, which is tucked underneath the overlap between the frontal and temporal lobes (Maffei, Haley, & Fontanini, 2012; Roper, 2013). There are even methods for quantifying individual differences in taste perception, which lead us to the detection of ‘supertasters.’ (Bartoshuk, et al., 2004)
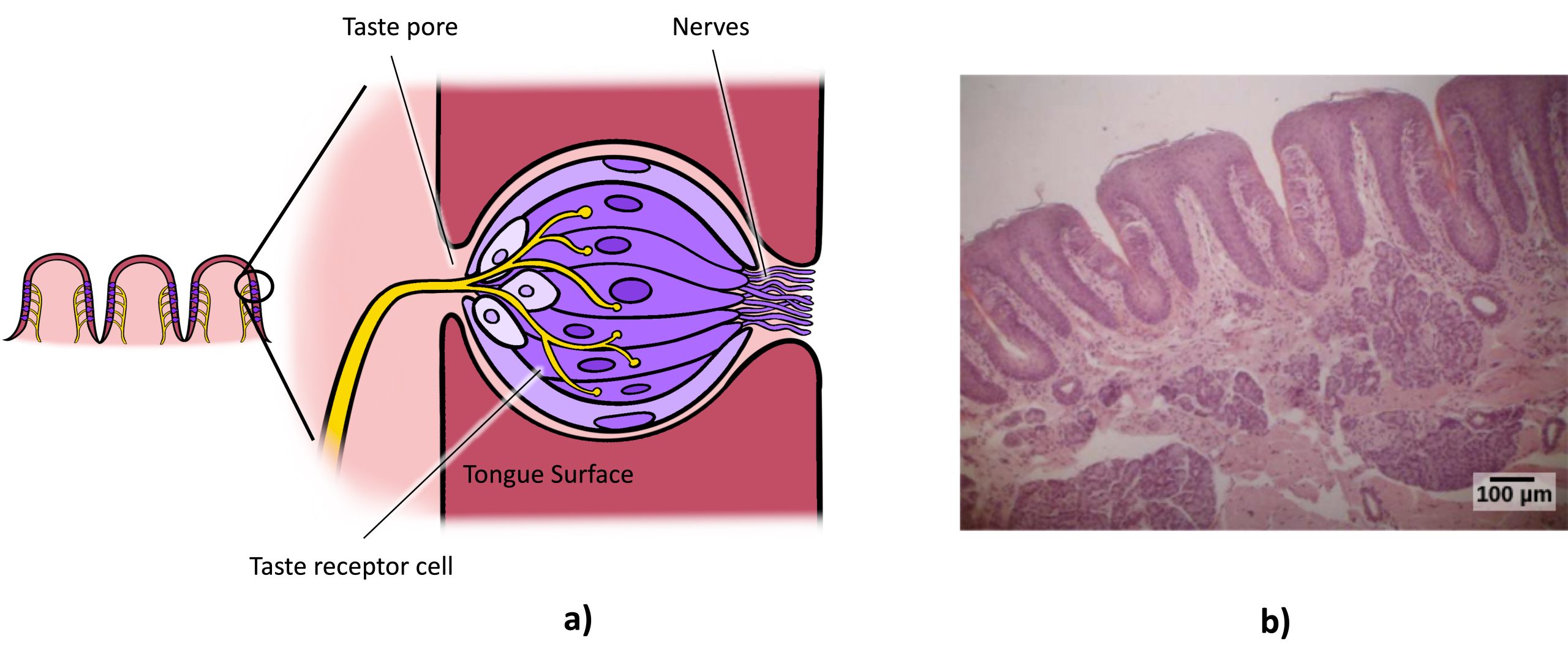
The concept of “supertasters” refers to individuals with an inherited heightened sensitivity to taste, particularly to the taste of certain bitter compounds. This heightened taste sensitivity can influence a wide range of taste-related behaviours and preferences, from food choices to brand loyalty in taste tests. Supertasters are characterised by having a higher density of taste papillae on their tongues, which contributes to their increased taste sensitivity. The phenomenon of supertasting suggests that genetic factors play a significant role in individual differences in taste perception, affecting not only dietary habits but also potentially marketing outcomes and product development strategies in the food and beverage industry (LaTour, Latour, & Wansink, 2017).
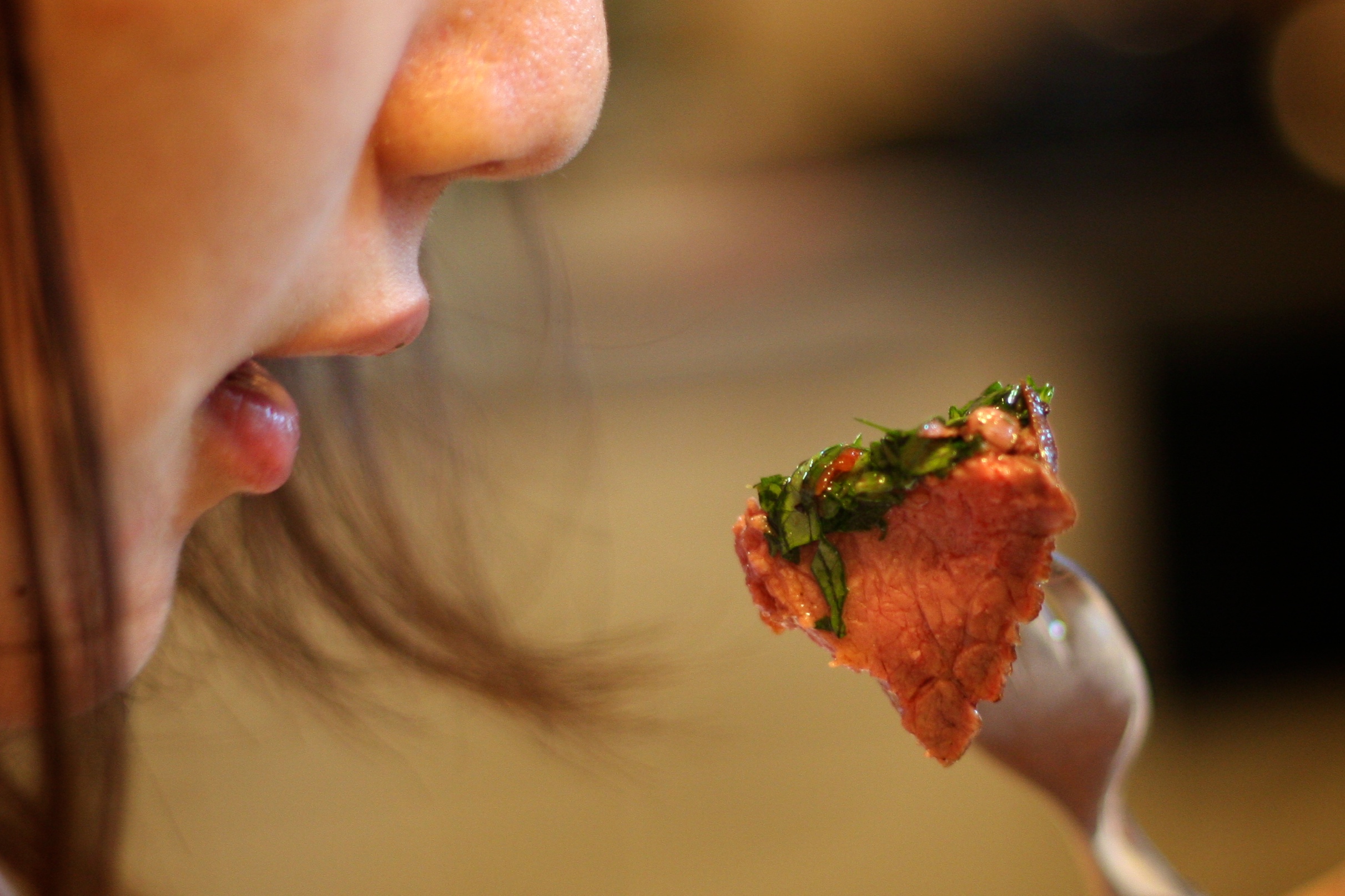
How you can tell if you are supertaster?
Here are some of the criteria used to detect super tasters (Hayes, & Keast, 2011):
- PROP Test: Supertasters can be identified using a test that compares the bitterness of a substance called PROP (6-n-propylthiouracil) to the saltiness of salt (NaCl). In this test, supertasters perceive PROP as much more bitter compared to how salty they find salt. This high level of bitterness is what sets supertasters apart.
- Intensity Ratings: Another way to identify supertasters is by asking them to rate how strong the bitterness of PROP is on a special scale. Supertasters will give very high ratings for the bitterness, indicating that they find PROP extremely bitter.
- Taste Bud Density: Although not the main method, people with a higher number of taste buds (specifically, those on the small bumps on their tongue called fungiform papillae) are often supertasters. These extra taste buds make them more sensitive to tastes, including the bitterness of PROP. Blue food dye is used to make taste buds more visible so their densities can be calculated. To measure the density of your fungiform papillae read the instructions in McMahon, 2007 (you will need blue food dye and a round loose leaf paper hole reinforcement sticker.
- Avoiding bitter foods. Supertasters avoid eating bitter vegetables and fruits.
Smell is another important way we understand the world. Just like taste, smell involves special cells that react to the things around us. These cells are in our nose and they help us recognise different smells. When we smell something, these cells send messages to our brain, telling us what we’re smelling. This is similar to how our taste buds work with taste. So, as we move from discussing taste to exploring smell, we’ll see how these two senses are different, but also how they work together to help us experience food and our environment.
Smell (Olfaction)
Olfactory receptor cells are located in a mucous membrane at the top of the nose. Small hair-like extensions from these receptors serve as the sites for odour molecules dissolved in the mucus to interact with chemical receptors located on these extensions (Figure SP.16). Once an odour molecule has bound to a given receptor, chemical changes within the cell result in signals being sent to the olfactory bulb: a bulb-like structure at the tip of the frontal lobe where the olfactory nerves begin. From the olfactory bulb, information is sent to regions of the limbic system and to the primary olfactory cortex, which is located very near the gustatory cortex (Lodovichi & Belluscio, 2012; Spors et al., 2013).
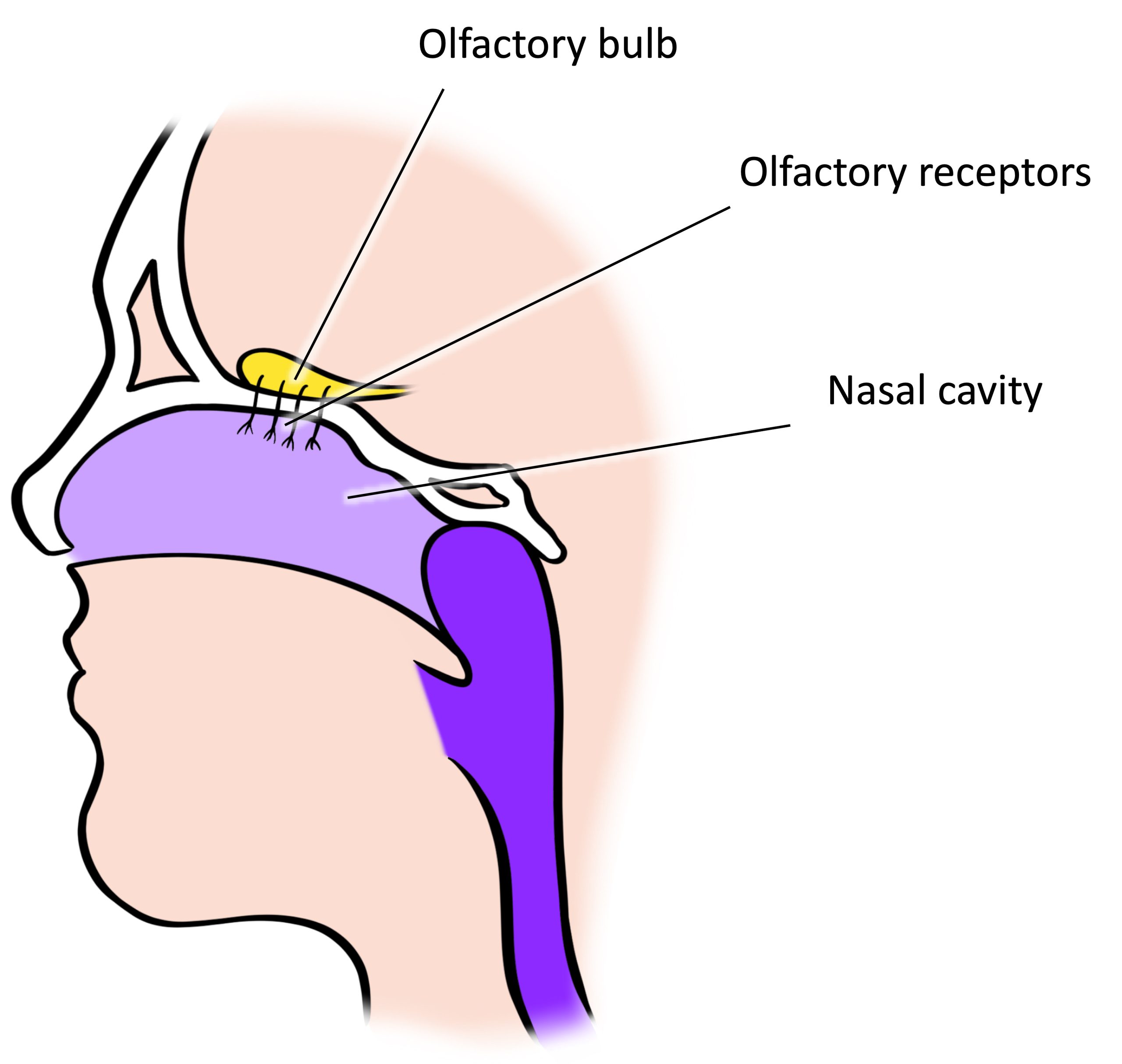
There is tremendous variation in the sensitivity of the olfactory systems of different species. We often think of dogs as having far superior olfactory systems than our own, and indeed, dogs can do some remarkable things with their noses. There is some evidence to suggest that dogs can “smell” dangerous drops in blood glucose levels as well as cancerous tumours (Wells, 2010). Dogs’ extraordinary olfactory abilities may be due to the increased number of functional genes for olfactory receptors (between 800 and 1200), compared to the fewer than 400 observed in humans and other primates (Niimura & Nei, 2007).
Many species respond to chemical messages, known as pheromones, sent by another individual (Wysocki & Preti, 2004). Pheromonal communication often involves providing information about the reproductive status of a potential mate. So, for example, when a female rat is ready to mate, she secretes pheromonal signals that draw attention from nearby male rats. Pheromonal activation is actually an important component in eliciting sexual behaviour in the male rat (Furlow, 1996, 2012; Purvis & Haynes, 1972; Sachs, 1997). Pheromones work in the same way across many species of animals.
The nose knows: Olfaction and brain health
Did you know that our sense of smell has a deep connection to our brain’s health and our cognitive abilities? As people grow older, they often find that their ability to detect scents starts to fade before they notice changes in their thinking skills (Doty et al., 1984). That’s not just a random observation; decreased olfaction has been linked to some notable changes in the brain, including reductions in both grey and white brain matter (Bitter et al., 2010a,b; Segura et al., 2013). More than just a nose issue, this decline in our sense of smell can sometimes give us early hints about bigger health concerns, like the onset of Alzheimer’s or Parkinson’s diseases (Conti et al., 2013; Ponsen et al., 2004).
Interestingly, olfaction also offers a pathway to intervention. Through ‘olfactory enrichment,’ which involves exposing individuals to a diverse range of scents, there’s potential not only to improve olfactory ability but also to induce positive changes in brain structure and function (Konstantinidis et al., 2013; Al Aïn et al., 2019; Gellrich et al., 2017; Han et al., 2021). The process, particularly when done at night, can influence key neural pathways like the uncinate fasciculus, crucial for memory and learning (Morikawa et al., 2010).
Cynthia Woo and her colleagues (2023) studied a group of participants, aged between 60 and 85, who were exposed to essential oil scents such as rose, orange, eucalyptus, lemon, peppermint, rosemary, or lavender for 2 hours every night or were part of a control group with minimal odour exposure (trace amounts of odourant). Over 6 months, the aim was to assess whether olfactory stimulation could influence cognitive performance. Cognitive assessments were carried out at the start of the study and after 6 months.
The research of Woo et al (2023) yielded remarkable results! The olfactory-enriched group demonstrated a 226% improvement in performance compared to the control group. To put that astounding improvement into perspective, we psychology researchers might be impressed to find a 50% increase with any given intervention. Woo and colleagues’ olfactory enrichment caused an effect more than four times that amount. Furthermore, brain imaging techniques in the Woo et al. (2023) study revealed significant changes in the left uncinate fasciculus among participants in the olfactory enrichment group. Validating that the olfactory enrichment specifically stimulated growth in brain cells.
How exactly does olfactory enrichment work on memory? Olfactory enrichment involves exposing the brain to various scents (one single scent per night and then changing the scent every day or so) which can enhance cognitive functions. This exposure triggers the release of noradrenaline, a chemical that aids in learning and attention. This process leads to brain plasticity, where new neural connections form, improving memory and cognitive flexibility. Essentially, smelling a range of scents can make the brain more efficient and adaptable, offering a simple method to support brain health, especially with ageing (Terrier et al., 2023).
These findings underscore the profound relationship between olfaction and cognitive health. Olfactory enrichment, particularly during the night, holds promise as a potential strategy to support cognitive functions and maintain optimal brain health. As we further our understanding of this relationship, it’s evident that our sense of smell offers more than mere sensory experiences; it’s intrinsically linked to the health and vitality of our brains.
Watch this video: Taste & Smell: Crash Course Anatomy & Physiology #16 (10.5 minutes)
“Taste & Smell: Crash Course Anatomy & Physiology #16” video by CrashCourse is licensed under the Standard YouTube licence.
Skin senses: Touch, Thermoception, and Nociception
A number of receptors are distributed throughout the skin to respond to various touch-related stimuli: mechanoreceptors, thermoreceptors, and nociceptors (Abraira & Ginty, 2013).
- Mechanoreceptors: These are specialised types of sensory receptors that are sensitive to mechanical pressure or distortion. They are found in various areas throughout the body, including the skin, bones, muscles, and internal organs. For example, when you touch a surface or feel vibration, mechanoreceptors in your skin detect these sensations. Mechanoreceptors also play key roles in proprioception, which is your body’s sense of its own position in space, and in the sensations of muscle stretching and force.
- Thermoreceptors: These receptors are responsible for detecting changes in temperature. They are found in the skin, as well as in other deeper tissues of the body, and even in certain organs such as the hypothalamus of the brain, which monitors body temperature. There are two kinds of thermoreceptors: ones that respond to cold (found in the epidermis, the outer layer of skin) and ones that respond to warmth (found a little deeper in the skin).
- Nociceptors: These are sensory receptors that are activated by harmful or potentially harmful stimuli. They can respond to extreme temperatures (hot or cold), high pressure or mechanical deformation, and chemical substances released from tissue damage. When nociceptors are activated, they send signals to the brain that are interpreted as pain.This helps to protect the body by alerting it to potential damage or injury.
A number of receptors are distributed throughout the skin to respond to various touch-related stimuli (Figure SP.17). These various receptors respond to: moderate pressure and lower frequency vibrations, transient pressure and higher frequency vibrations, light pressure, and stretching (Abraira & Ginty, 2013).
In addition to the receptors located in the skin, there are also a number of free nerve endings that serve sensory functions. These nerve endings respond to a variety of different types of touch-related stimuli and serve as sensory receptors for both thermoception (temperature perception) and nociception (a signal indicating potential harm and maybe pain) (Garland, 2012; Petho & Reeh, 2012; Spray, 1986). Sensory information collected from the receptors and free nerve endings travels up the spinal cord and is transmitted to regions of the medulla, thalamus, and ultimately to the somatosensory cortex, located in the parietal lobe.
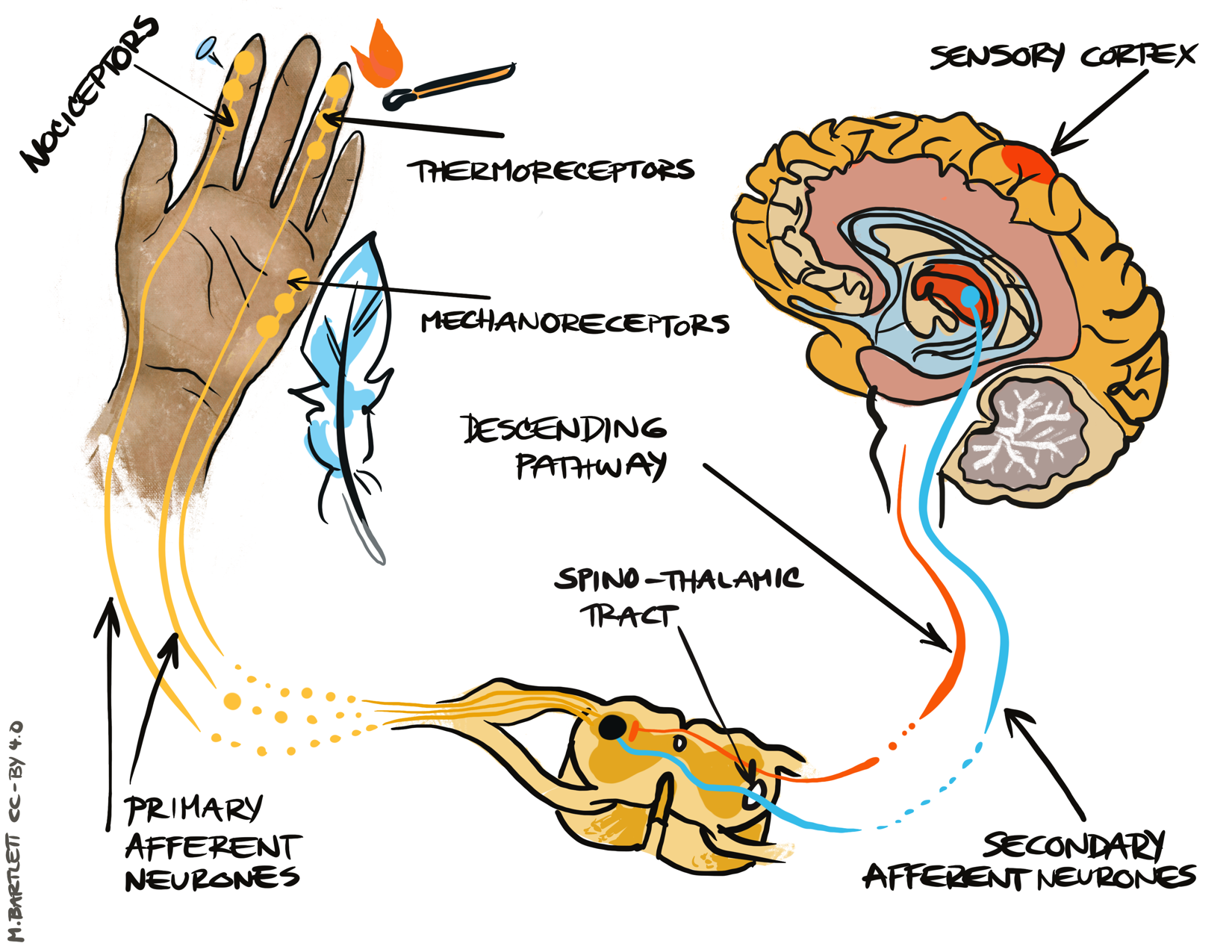
Watch this video: Types of Sensory Receptors (7.5 minutes)
“Types of Sensory Receptors” video by Drbeen Medical Lectures is licensed under the Standard YouTube licence.
Pain Perception
Pain is an unpleasant experience that involves both physical and psychological components. It plays a vital role, serving as a biological alarm system to notify us of potential harm or existing injury. The experience of pain can also discourage additional injury by prompting us to be cautious or reduce movement in the affected area (Raja et al., 2020; Aydede, 2020; De Ridder, et al., 2021; Love-Jones, 2019; Del Sordo, et al., 2020).
Pain is generally categorised into two types: inflammatory and neuropathic. Inflammatory pain is often acute, acting as the immediate response to tissue damage, which can be associated with injuries to the body (somatic: e.g., a sprained ankle or a tooth infection) or organs (visceral: e.g., appendicitis or tonsillitis). In contrast, neuropathic pain usually stems from an injury to, or dysfunction within, the nervous system (e.g., sciatica or shingles). This neuropathic pain leads to a state where pain signals are amplified and pain can be felt even without a specific trigger (Costigan, Scholz, & Woolf, 2009). Indeed, Pustilnik (2019) advocates that we consider chronic pain a sensory disability. She explores how understanding pain in this way can inform legal and moral perspectives.
Management strategies for pain are diverse due to its physical and psychological complexity. These can range from non-pharmacological interventions such as physical therapy and mindfulness-based therapies, to drug treatments. Medications like opioids, non-steroidal anti-inflammatory drugs (NSAIDs), and certain antidepressants and anticonvulsants are commonly used for pain relief (Turk, Wilson, & Cahana, 2011).
The complexities of pain perception and its management play significant roles in our health, often becoming intertwined with mental health conditions and leading to substance use disorders. The alarming rise in opioid-related deaths across Canada serves as a stark reminder of the profound societal and public health implications of opioid misuse. It is vital for us to understand the mechanisms of pain, the interactions between pain, mental health, and substance use, as well as the potential life-saving role of interventions such as naloxone. See the Well-being chapter for more on this topic). This understanding is the first step in developing effective strategies to combat the current opioid crisis, provide help to those in need, and ultimately save lives.
The senses of 3D space: Vestibular sense and proprioception
The vestibular sense contributes to our ability to maintain balance and body posture. As Figure SP.18 shows, the major sensory organs (utricle, saccule, vestibule, and the three semicircular canals, horizontal, posterior, superior) of this system are located next to the cochlea, basilar membrane, and hair cells in the inner ear. The vestibular organs are fluid-filled and have hair cells, similar to the ones found in the auditory system, which respond to movement of the head and gravitational forces. When these hair cells are stimulated, they send signals to the brain via the vestibular nerve. Although we may not be consciously aware of our vestibular system’s sensory information under normal circumstances, its importance is apparent when we experience motion sickness and/or dizziness related to infections of the inner ear (Khan & Chang, 2013).
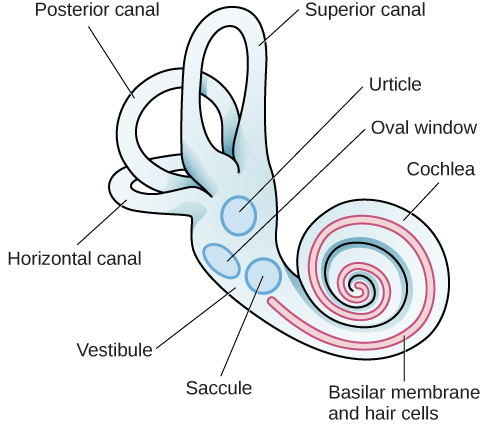
Here is a mnemonic to help you memorise the anatomy of the inner ear.
“Under Violet Skies, Hairy Squirrels Perform Outrageous Wild Cartwheels, Ballet Moves, and Happy Calls”
- Under (Utricle)
- Violet (Vestibule)
- Skies (Saccule)
- Hairy (Horizontal Canal)
- Squirrels (Superior Canal)
- Perform (Posterior Canal)
- Outrageous Wild (Oval Window)
- Cartwheels, (Cochlea)
- Ballet Moves, (Basilar Membrane)(and)
- Happy Calls (Hair Cells)
In addition to maintaining balance, the vestibular system collects information critical for controlling movement and the reflexes that move various parts of our bodies to compensate for changes in body position. Therefore, both proprioception (perception of body position) and kinesthesia (perception of the body’s movement through space) interact with information provided by the vestibular system.
These sensory systems also gather information from receptors that respond to stretch and tension in muscles, joints, skin, and tendons (Lackner & DiZio, 2005; Proske, 2006; Proske & Gandevia, 2012). Proprioceptive and kinesthetic information travels to the brain via the spinal column. Several cortical regions in addition to the cerebellum receive information from and send information to the sensory organs of the proprioceptive and kinesthetic systems.
Interoception
Interoception is our ability to perceive internal bodily sensations such as hunger or changes in heart rate. This capability is fundamental to self-awareness and significantly influences both our physical and mental health. This idea, first put forth by Sherrington [1906] and developed by Craig [2002; 2007], demonstrates how bodily signals like hunger and pain have a significant impact on our emotions and daily interactions. How we detect, interpret, and respond to these internal cues can shape our emotions and interactions with the environment [Garfinkel et al., 2015; Parker, 2022a]
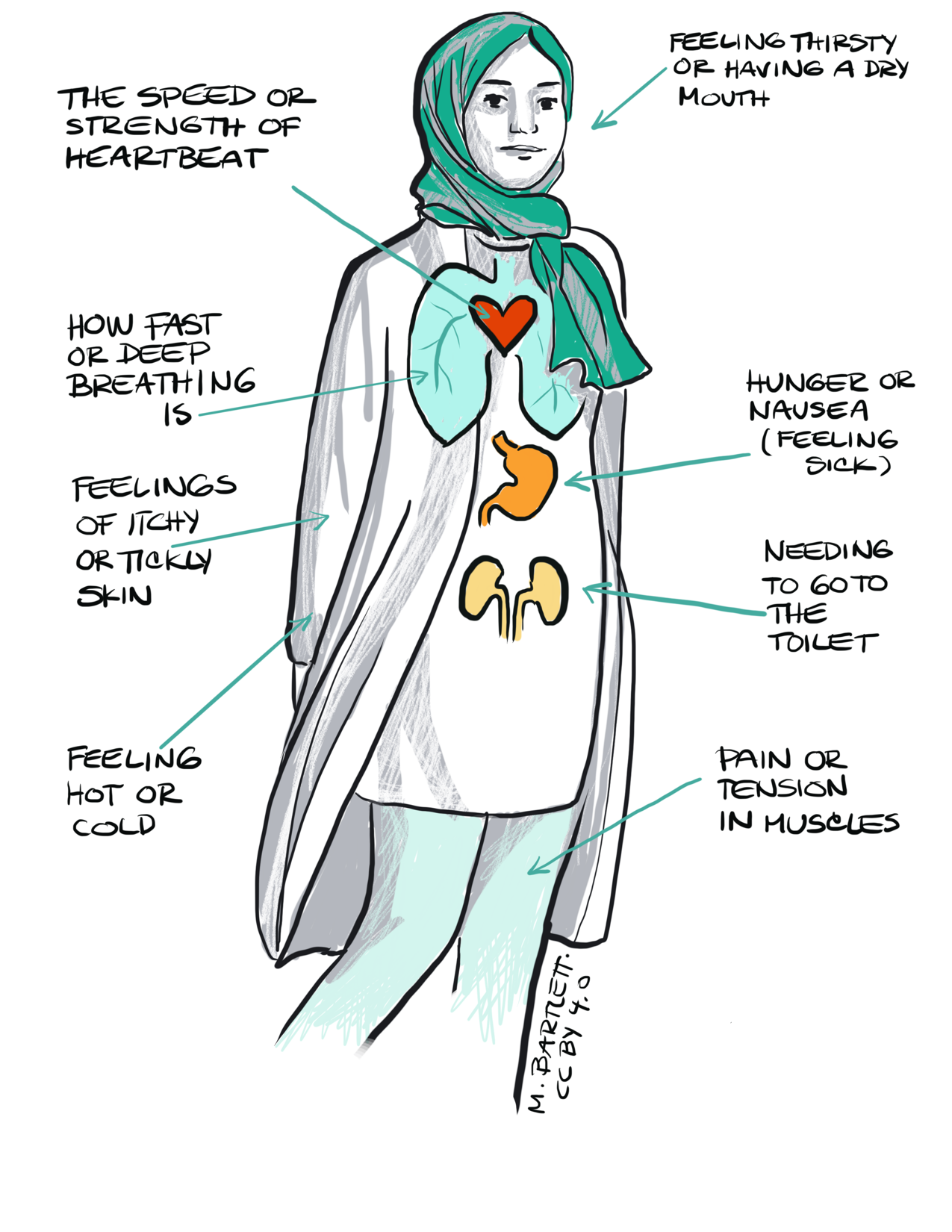
Why should we learn about interoception?
Understanding interoception is essential because it impacts our physical, emotional, and mental well-being. Recognising and responding to internal bodily signals is vital for our health, and impaired interoceptive awareness is linked to mental health disorders such as depression and anxiety [Duquette, 2017]. Therefore, it is important for clinicians to assess clients’ interoceptive awareness during mental health evaluations and to consider clients’ interoceptive strengths and weaknesses when planning psychotherapeutic treatments.
Interoception helps us recognise and respond to internal bodily signals, such as hunger, thirst, or changes in heart rate. If our interoceptive awareness is impaired, it may contribute to mental health disorders, including depression and anxiety (Duquette, 2017). For example, when we can’t accurately sense and understand our increased heart rate, we might misinterpret it as a sign of danger or panic, even if there isn’t a real threat. This misunderstanding can make us feel more stressed and anxious, potentially leading to an anxiety episode because our brain thinks something is wrong based on the confusing signal from our body. Teach clients to improve their sense of interoception can be an important component of mental health evaluations and psychological treatments.
Mindfulness and similar practices significantly enhance our ability to understand and respond to internal bodily signals (Farb et al., 2015). Initially, our perceptions of these signals might be biased and inaccurate, affecting our emotional responses in ways we might not fully realise.
By engaging in mindfulness, we can correct these biases and achieve a more accurate understanding of our bodily sensations (Farb et al., 2015). For example, noticing when your heartbeat quickens or you start sweating could indicate anxiety or nervousness. Recognising and understanding these signs is crucial for managing them effectively.
As we become more attuned to these internal cues, we improve our emotional awareness and control. This awareness allows us to connect physical sensations to emotions, helping us identify what triggers our responses (Aspell & Todd, 2023). For instance, if you recognise that social settings accelerate your heartbeat, indicating anxiety, you can prepare by using relaxation techniques or reframing your thoughts about these situations (Duquette and Ainley, 2019).
Instead of acting impulsively as a result of unchecked emotions, you can make more thoughtful decisions about how to act or respond, thanks to this improved understanding of your emotions. In therapy, this capability allows individuals to discuss their feelings more precisely and work through them constructively, leading to meaningful changes and better emotional health (Aspell & Todd, 2023; Duquette & Ainley, 2019). Such improved emotional comprehension and control can facilitate significant therapeutic progress.
How do we experience interoception?
Here are several ways we can experience interoception:
- Interoceptive awareness: This is when we can consciously notice things happening inside our body. For example, feeling your heart beat faster when you’re nervous, like before speaking in front of people (Pollatos & Schandry, 2008).
- Interoceptive coherence: This is about how well our physical feelings match up with what we think we’re feeling. Like when you’re grumpy because you’re hungry, even if you don’t realise you’re hungry (Garfinkel et al., 2015).
- Interoceptive attention tendency: This is how much we notice and think about certain inner feelings. For example, someone might constantly be aware of how their stomach feels to decide if they need to eat or if they’re getting sick. This means they’re really paying attention to this specific type of body signal (Mehling, 2016).
- Interoceptive sensitivity: This is about how quickly we notice changes in our body. Some people can feel even small changes in their heartbeat when they exercise (Garfinkel et al., 2015).
- Interoceptive accuracy: This is how well we can identify specific feelings from all the other things we’re feeling. For instance, noticing that a fast heartbeat is due to feeling anxious about an upcoming test, rather than because you just ran up some stairs (Garfinkel et al., 2015).
- Interoceptive sensibility: This is about how well a person can feel and be certain about their inner sensations. For example, someone who can recognise that they are tired and need to stop working for the day because they are no longer able to concentrate has a high interoceptive sensibility (Mehling et al., 2018).
- Interoceptive regulation: This is our ability to change our internal feelings to match what we want to feel, like using deep breathing to calm down when you’re feeling panicked (Duschek et al., 2015).
Interoception is like having a friend who helps you better understand your feelings and circumstances. It’s the racing of your heart when you’re excited, the butterflies in your stomach before something big, and the warmth you feel in happy moments. These feelings are your body’s way of talking to you. Listening to these signals can help you make better choices, enjoy life more, and connect with others. So, take a deep breath now and listen. What is your body saying to you? This inner conversation is one of the most valuable ones you’ll ever have (Parker, 2022).
Image Attributions
Figure SP.14. Figure SAP.21 (a) created by Molly Wells Art as found in Introduction to Psychology & Neuroscience (2nd Edition) is licensed under a CC BY 4.0 License and foliate papillae is in the public domain.
Figure SP.15. Image credit: “Eat!” by Joshua Rappeneker is licensed under CC BY-SA 2.0.
Figure SP.16. Figure SAP.22 created by Molly Wells Art as found in Introduction to Psychology & Neuroscience (2nd Edition) is licensed under a CC BY 4.0 License.
Figure SP.17. Skin senses by Marie Bartlett is licensed under a CC BY 4.0 license.
Figure SP.18. Figure 5.24 as found in Psychology 2e by OpenStax is licensed under a CC BY 4.0 License.
Figure SP.19. Interoception by Marie Bartlett is licensed under a CC BY 4.0 license.
To calculate this time, we used a reading speed of 150 words per minute and then added extra time to account for images and videos. This is just to give you a rough idea of the length of the chapter section. How long it will take you to engage with this chapter will vary greatly depending on all sorts of things (the complexity of the content, your ability to focus, etc).