Learning Task 4
Calculating Transmission Loss
Rooms have ceilings, floors, and walls with doors and windows. Transmission losses must be calculated for each surface that has unconditioned space on the other side of it. This means that a floor with a heated room below it would be considered to not have any downward heat loss. The same is said for a ceiling with a heated room above it or a wall that abuts a heated room. In short, only surfaces that separate a heated space from the outdoors need to have heat losses calculated for them.
Transmission Losses through Windows and Doors (Openings)
The “U” values used for doors are the same as for windows. A “U” value, based on parameters such as single, double or triple glazed with or without special coatings and fillers, is selected from a table, and multiplied by area in ft² and by the DTD. As an example, 48 ft² of doors and windows with a “U” value of 0.59 in Penticton would calculate to be 48 × 0.59 × 67 = 1,897 BTUH.
Walls and Openings
Walls that separate heated (conditioned) spaces from the outdoors are called “exposed walls”, and they will have an area that includes windows and doors. The losses through windows and doors (“openings”) must be calculated separately, as they will have different “U” factors than will the walls themselves (see above). The easiest way to perform an exposed wall calculation is to do a takeoff of the area of the openings first. If a room has a total of 108 ft² of openings in its exposed walls, that area would be subtracted from the total area (length × height) of the exposed wall. So, an exposed wall that measures 28 feet long by 8 feet high would have a gross area of 224 ft². When the openings are subtracted from that gross area, the net area of exposed wall becomes 224 – 108 = 116 ft². The heat loss formulas can now be applied to the net wall area. Table 2 lists the “U” values for framed walls whose exterior finishes are lumped together into one category. In other words, it doesn’t matter what the exterior wall finish is – the “U” value depends upon the “R” value of the insulation in the wall cavities. So, for our exterior wall in Penticton, with a net area of 116 ft², using R20 insulation in the walls, the calculation would be 116 × 0.05 × 67 = 389 BTUH
Ceiling and Floor Losses
Ceiling losses will only be counted for rooms on a top floor, and are listed as either “roof with attic space” (normal residential construction using trusses) or “built up roof, no attic space” (typically flat or vaulted roofs) with an insulation value attached to them. Floor losses (other than basements) will only be counted if they are located over unheated space such as an open carport (“wood over exposed space”) or a garage (“wood over enclosed space”). The insulation values will determine the “U” value chosen from the table, and their transmission losses are calculated in the same manner as with openings and exposed walls.
Thermal Bridging
Our previous example did not make mention at all of a thermal resistance for the 2 × 6 studs themselves. This is because they are spaced at either 16″ or 24″ centres along the wall and are difficult to allow for. Some heat load designers will include a small allowance for the studs when coming up with the “U” value for an assembly but this is a matter of designer preference.
The studs that form the support system for walls will have a heat loss through them which is higher than that of the insulation between them. This occurrence is known as “thermal bridging”. By definition, thermal bridging, also known as cold bridging or thermal bypass, is an area or component of an object which has a higher thermal conductivity than the surrounding materials, creating a path of least resistance for heat transfer. Overlooked for a great period of time, thermal bridging has recently come to the forefront of heat load calculations as designers strive to be as accurate and comprehensive as possible in their estimates. Thermal bridging not only causes a loss of heat within the space, it can also cause the warm air inside the space to cool down. When heat attempts to escape a room, it follows the path of least resistance. Likewise, the same process occurs during the summer, only in reverse, allowing heat to enter your otherwise cool building, called heat gain. Thermal bridging happens when a more conductive material allows an easy pathway for heat flow, usually where there is a break in (or penetration of) the insulation. Some common locations include:
- The junctions between the wall and the floor, roof, or doors and windows.
- The junction between the building and the deck or patio
- Penetrations in the building envelope to include pipes or cables
- Wood, steel, or concrete envelope components such as foundations, studs, and joists
- Recessed lighting
- Window and door frames
- Areas with gaps in insulation
In short, any area where there isn’t a continuous, unbroken layer of insulative material can be considered a thermal break. These areas should be addressed in both the design and construction phase, as studies have show that in an otherwise airtight and insulated home, thermal bridges can account for a heat loss of up to 30%. Whether you’re building a new home or retrofitting an existing structure, care should be taken to avoid unnecessary breaks or penetrations in the building envelope so that the possibility of thermal bridging decreases.
Slab Edge Loss
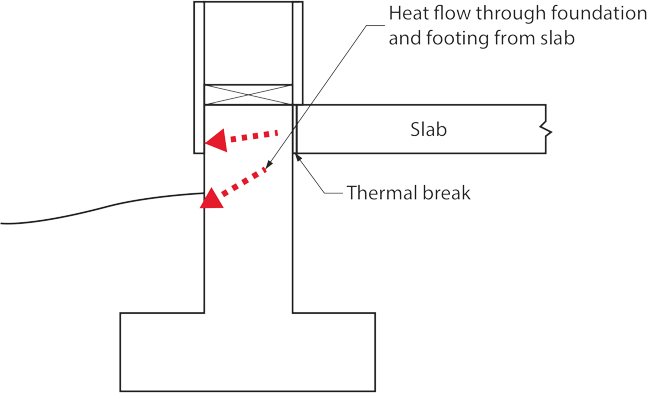
The thermal bridging that occurs at the point where the indoor concrete slab meets the concrete foundation wall is almost inevitable. Concrete has a low “R” value and there is little that can be done to mitigate the transmission of large amounts of heat between the two masses. Therefore, there will be a category of heat loss called “slab edge loss” that is calculated when using slab-on-grade construction. The formula is written as:
Slab edge length (ft) × “U” value × ΔT = BTUH.
The slab edge “U” factor, from the table above, is either 0.69 when using 1” insulation, or 0.53 when 2” insulation is used.
As an example, if a room with slab-on-grade construction had 32 lineal feet of exposed slab edge with 2” insulation and the DTD was 67°F, the calculated slab edge loss would be 32 × 0.53 × 67 = 1,136 BTUH. This would be added to the transmission and infiltration losses for that room.
With radiant floor heating, slab edge insulation is a must. A strip of rigid insulating material such as polystyrene is sandwiched between the two concrete masses to try to reduce the thermal bridging effect. As well, rigid polystyrene (XPS) is commonly attached to the outside of the foundation wall, with a protective sheet of galvanized sheet metal covering it, to further reduce the heat loss through the concrete to the soil and outside air.
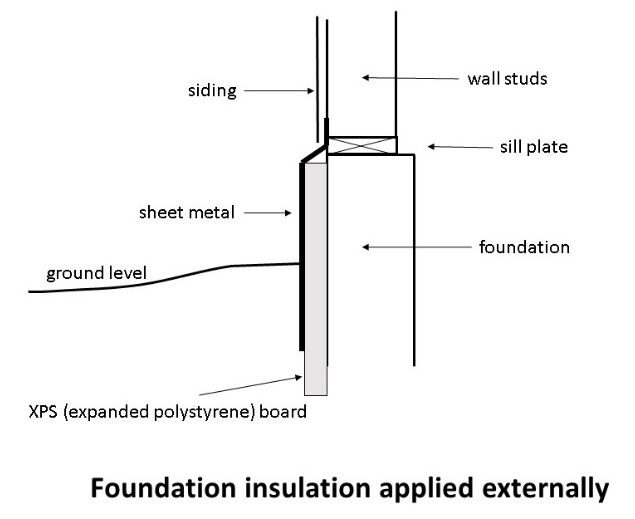
Basement Concrete Slab Loss
If a basement concrete floor is more than 2 feet below finish grade, a downward loss should be calculated. The formula is similar to that for walls, except that the IDT can be lower if desired. Using 65°F (18°C) as an allowable value for an unfinished basement, the calculation would be:
Area (ft²) × DTD (65 − ODT) × 0.04 (“slab below grade” from “U” values table above) = BTUH
If the slab is being heated through radiant means, then the slab temperature is used as the IDT.
Infiltration/Exfiltration Loss
Today’s structures are far more airtight than were their counterparts of 50 years ago. Old wood frame double-hung, single pane windows have long ago given way to double or triple glazed gas-filled reflective windows mounted in vinyl frames. Caulking, weatherstripping and energy-conserving measures make today’s structures far less drafty than ever before. That said, there are still imperfections in building envelopes that will allow heated interior air to escape to the outdoors, or cold outside air to be forced into the living space. These heat losses, which can be classified as due to convection, are simply referred to as “infiltration losses”.
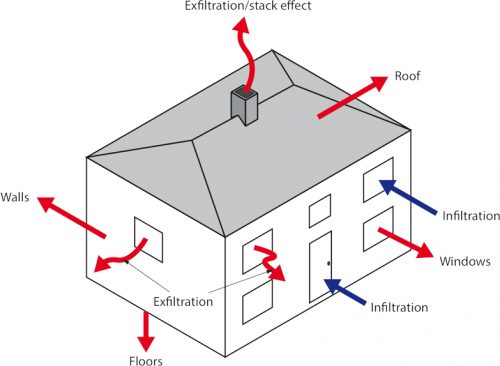
Heat loss from infiltration (inward flow) and exfiltration (outward flow) is uncontrolled air leakage through joints in the construction and cracks around windows and doors. In the winter, the cold air that infiltrates the building is equal to the amount of hot air that escapes. Conversely, in the summer, the cooler air inside escapes and hot exterior air infiltrates. Infiltration is caused by wind and stack-driven pressure differentials, which prompt air movement within the building envelope. In British Columbia the BC Building Code sets out requirements that are meant to both offset infiltration losses while also delivering fresh air for the health of occupants. The infiltration rate varies greatly depending on climate and the tightness of construction. In general, ASHRAE (American Society of Heating, Refrigeration and Air Conditioning Engineers) recommends for small buildings with low infiltration rates (<0.5 air changes per hour, known as “tight” or “airtight” construction) to use mechanical ventilation to ensure good indoor air quality (IAQ). We’ll confine our focus to the determination of the heat lost through infiltration rather than the requirements for mechanical air exchange.
Calculating Infiltration Loss
Just as in the calculation of transmission loss, there is a formula used for calculating losses of heat through infiltration, and it is based upon the fact that air has a specific heat, by volume, of 0.018 as mentioned earlier in the heading “water vs air as a heating medium”. So, the formula is written as:
Infiltration loss = ACH (# of air changes per hour) × 0.018 (specific heat of air) × DTD ( design temperature difference) × room volume (ft³).
The determination of an air change rate for a room is somewhat arbitrary, in that there are many factors at play. For our purposes within this learning guide, we will use the Air Change Table found in TECA’s literature below as our benchmark.
Description | Infiltration Rate | AC Factor* |
---|---|---|
R2000 Dwellings ONLY w/fully distributed ventilation systems (HRV’s) | [latex]\dfrac{1}{3}[/latex] ACH (air change/hr) | .005 |
Rooms w/windows on 1 side only | [latex]\dfrac{1}{2}[/latex] ACH | .009 |
Rooms w/windows on 2 sides | [latex]\dfrac{2}{3}[/latex] ACH | .012 |
Rooms w/windows on 3 sides | 1 ACH | .018 |
Entrance Halls | 1 ACH | .018 |
Sum rooms w/windows or doors on three sides | [latex]1\dfrac{1}{2}[/latex] ACH | .027 |
Rooms w/fireplace (except sealed combustion units_ | 1 – [latex]1\dfrac{1}{2}[/latex] ACH | .018 – .027 |
*[latex]\text{Air Change Factor}=\dfrac{\text{Infiltration Rate}}{60}\times 1.08[/latex]
[latex]\text{For Example:} \dfrac{\frac{1}{2} \text{ACH}}{60} \times 1.08 = .009[/latex]
As shown in the air change table above, air change rates vary from [latex]\dfrac{1}{3}[/latex] air changes per hour (ACH) to 1 ½ ACH. The choice of which one to use for a room comes down to determining how many exterior walls in that room have openings (doors or windows) and the type of room it is. For example, if a corner bedroom has two exterior walls but only one of them has windows, it would be classified as having ½ ACH. If the bedroom were 8 ft × 10 ft × 8 ft high, its volume would be 640 ft³. If ½ of the room’s air volume needed to be reheated every hour, we would need to heat 320 ft³ of that room’s air. Rather than split a room’s volume into [latex]\dfrac{1}{3}[/latex], ½, etc., we instead leave the room volume alone and adjust the specific heat value for air which is shown in the far-right column. What would otherwise have been a calculation of (640 ft³ × [latex]\dfrac{1}{2}[/latex]) × 0.018 × DTD = BTUH infiltration loss, would then become 640 ft³ × (0.018 × 0.5) × DTD = BTUH infiltration loss. The net result is the same, however the specific heat value is altered to be a multiplier instead of using only a portion of the room’s volume. This also makes it easier for use in the formulas that are in use within the TECA heat loss software programs.
An entrance hall, which is a room with an outside door that is being used frequently, would have a higher rate of air exchange than, say, a bedroom. It is listed at 1 ACH. Solariums (sun rooms), with a lot of glass area, and rooms with wood-burning fireplaces, have an even greater expectation of exfiltration loss, so are listed as 1 ½ ACH.
As you can see from Table 3 above, the determination of which factor to use when performing an infiltration loss using the TECA guidelines is simply reduced to identifying how many exposed walls in that room have openings (doors or windows) and what type of space it is.
Additional Heat Losses
Hot water heating systems may be designed to provide heat for other services or equipment such as:
- domestic hot water
- swimming pool
- hot tub
- outdoor radiant panels for melting snow
The heat loss for these services and equipment must be determined in an appropriate manner, and in some but not all cases added to the total. A swimming pool is a good example of not having its heat load added to that of the building because, in most cases, swimming pools are shut down and not used in the heating season. The energy needed for heating of the domestic hot water can be prioritized over the heat needed for the building, using the rationale that for the period of time that the heating plant is directing energy to the domestic water, the building can “coast” on its retained heat. In this way, the domestic water can be heated instead of the building, so that there is no additional heat needed.
A hot tub’s heat load would certainly be added to that of the building because they are often used and enjoyed more in the heating season, and the need to add a snow melting system’s load onto that of the building is also obvious.
Calculation of the loads for swimming pools, hot tubs and snow melt will not be part of this learning package.
Oversizing the Heating System
There are many conflicting thoughts on determining the size of the heating plant and components. Some heating professionals will add 15 to 30% to the heat load to compensate for other losses within the system, such as heat loss through the piping (“piping tax”) and additional capacity required for start-up of a cold system (“warm-up allowance” or “pickup allowance”). The extra capacity also guarantees sufficient heat if the outdoor temperature drops below the ODT, if use patterns cause additional heat losses, and if the residents prefer a temperature above the IDT.
Other professionals claim that these extra losses are minimal and that the heating system rarely runs at design conditions, so it is already in fact oversized. This rationale results in a lower installation cost. Those professionals would allow for 100% of the calculated heat load for the building and no more when selecting a heat plant. Also keep in mind that, no matter which sizing rationale is used, boiler inputs normally increase in increments of 25,000 to 50,000 Btuh, so slightly oversizing the boiler is almost inevitable.
Now complete the self test below.
Self-Test 1
Self-Test 1
- Which one of the following is not a method of heat loss found in building heating systems?
- Conduction
- Respiration
- Radiation
- Convection
- What is the approximate percentage of heat that a human body loses by radiation?
- 48
- 30
- 22
- 12
- What is the phenomenon known as, whereby a person can feel cold because they are sitting too close to a window or exterior door, when the temperature setting in the room is normal?
- “Warm 98.6”
- “Cold 98.6”
- “Warm 70”
- “Cold 70”
- What is the name given to the set of interior room temperature conditions where the human body feels the most comfort?
- The desired setpoint
- The ideal heat curve
- The desired heat curve
- The ideal setpoint
- What is the temperature that is read on a thermometer indicating?
- The quantity of heat present
- The latent heat present
- The intensity of heat present
- The BTUs that are present
- Which one of the following formulas would be used to calculate the number of BTUS involved in the heating of water within a heating plant, such as a boiler?
- BTUS = Mass × ΔT × S.H.
- BTUS = Mass × latent heat × ΔT
- BTUS = Latent heat × ΔT × area
- BTUS = Area × ΔT × S.H.
- What is the ΔT used in the design of most hot water heating systems that are fed from a boiler?
- 140°F
- 90°F
- 20°F
- 5°F
- What is the minimum return water temperature that should be maintained when using a non-condensing boiler?
- 20°F
- 90°F
- 120°F
- 140°F
- What is the term known as whereby heat is able to be applied to only the areas of a building where it is needed, rather than to the whole building?
- Zoning
- Priority
- Area selection
- Heat propagation
- What would be the “K” value for a material that has an insulating value of “R” = 22?
- 23
- 22
- 11
- 0.045
- How many BTUs would be involved in the transfer of heat through 150 ft² of a material that has an “R” value of 14 if the temperatures on either side of the material are 72°F and 5°F?
- 704
- 809
- 140,700
- 161,700
- When designing a heating system, what is the “January 2.5%” value for that geographical area known as?
- The required temperature
- The estimated temperature
- The indoor design temperature
- The outdoor design temperature
- According to the TECA Hydronic System Guidelines, what is an allowable IDT that can be used residentially when performing a heat loss estimate if hydronic radiant panels are intended as the primary source of heat?
- 140°F
- 80°F
- 72°F
- 68°F
- According to Part 9 of the BCBC, what is the allowable IDT for an unfinished basement?
- 15°C (59°F)
- 18°C (64°F)
- 20°C (68°F)
- 22°C (72°F)
- In heat loss language, what is the term for heat that is lost from a building through conduction?
- Radiation
- Infiltration
- Convection
- Transmission
- What is the term for heat that is lost when cold air makes its way into the building through cracks and imperfections in the building’s envelope?
- Radiation
- Infiltration
- Conduction
- Transmission
- Using Table 1, calculate the “U” value for an exterior wall made up of outside air film on 4″ brick on ½″ plywood sheathing on 2 × 6 wood studs with fiberglass batt insulation on vapour barrier on ½″ gypsum wall board on inside air film (use average value for fiberglass batt insulation).
- 0.04
- 0.16
- 0.52
- 22.83
- According to Table 2 (Heat Loss U-Factors Table), which one of the following double-glazed windows would have the best insulation rating?
- [latex]\dfrac{1}{4}[/latex]″ air space
- [latex]\dfrac{1}{2}[/latex]″ air space
- [latex]\dfrac{5}{8}[/latex]″ air space
- Low “E” – argon filled
- What is the term given to the situation where, for instance, a heated slab-on-grade butts up to the exterior foundation wall, allowing heat to transfer between the two materials more easily than through the surrounding structure?
- Infiltration
- Convection
- Thermal bridging
- Thermal connectivity
- What would be the infiltration loss for a corner bedroom that measures 20 ft × 15 ft × 8 ft, has openings on both exterior walls, with an ODT of 5°F and an IDT of 72°F?
- 1,447 BTUH
- 1,930 BTUH
- 2,894 BTUH
- 4,342 BTUH
- What would be the expected outcome of the rate of heat transfer if the ΔT between two sides of a wall or ceiling were doubled?
- The rate would be halved
- The rate would stay the same
- The rate would double
- What would be the expected outcome of the rate of heat transfer if the “R” value of a material were doubled?
- The rate would be halved
- The rate would stay the same
- The rate would double
- A contractor plans to build two identical houses, one in Inuvik and the other in Vancouver. Which one of the following statements would be true?
- The Vancouver house’s heat loss will be the greater of the two.
- The Inuvik house’s heat loss will be the lesser of the two.
- The IDT for the two houses will be different.
- The ODT for the two houses will be different.
- How many air changes per hour (ACH) would a room with openings on 3 sides undergo?
- [latex]\dfrac{1}{3}[/latex]
- [latex]\dfrac{1}{2}[/latex]
- 1
- [latex]1\dfrac{1}{2}[/latex]
- What is the “U” value to be used for a framed above-grade wall with stucco veneer with building paper, ½″ wood sheathing, studs, ½″ drywall and R14 insulation?
- 0.05
- 0.06
- 0.07
- 0.50
- What is the “R” value of 3 inches of extruded polystyrene?
- 15.0
- 10.0
- 5.0
- 4.0
- What is the air change factor to be used for an R2000 dwelling with a fully distributed ventilation system (HRV)?
- [latex]\dfrac{1}{3}[/latex] ACH
- [latex]\dfrac{1}{2}[/latex] ACH
- [latex]\dfrac{2}{3}[/latex] ACH
- 1 ACH
- What is the ODT to be used when designing a building in Youbou, BC?
- 22°C
- 15°C
- 22°F
- 15°F
- Calculate the heat loss (transmission and infiltration) for a bedroom above a carport if:
- the room is 12 feet long × 11 feet wide × 8 feet high
- there is a 3′ × 5′ window with double pane glazing with [latex]\dfrac{1}{2}[/latex]″ air space mounted in each of the two exterior walls
- walls are stucco with R20 insulation
- the floor beneath the bedroom has R20 insulation
- the attic space has R40 insulation
- the home is being built in Kimberly, BC.
- What would be the downward loss through a concrete floor that is 8 feet below finish grade if the house is in Powell River and the slab measures 17′ – 6″ wide by 33′ – 3″ long? (Assume an IDT of 65°F).
- 1,164 BTUH
- 1,327 BTUH
- 1,732 BTUH
- 1,614 BTUH
Check your answers using the Self-Test Answer Keys in Appendix 1.
Media Attributions
- Figure 1 Slab edge loss by ITA is licensed under a CC BY-NC-SA licence.
- Figure 2 Slab edge insulation by ITA is licensed under a CC BY-NC-SA licence.
- Figure 3 Heat loss via conduction and infiltration/exfiltration by ITA is licensed under a CC BY-NC-SA licence.