Learning Task 2
The Concept of Heat and Heat Transfer
Heat Loss (“Load”) Calculations
A btu (British Thermal Unit) is the quantity of heat required to raise the temperature of one pound of water by one degree Fahrenheit. Therefore, it would require adding 2 btus to 2 pounds of water in order to raise its temperature by 1°F, 3 btus for 3 pounds of water through 1°F and so on. The quantity of heat in btus needed for any process that involves the sensible heat that changes the temperature of an amount of water can be calculated by using this formula:
BTUs = mass × ΔT × S.H.
Mass will be the weight of water in pounds, ΔT will be the desired change in temperature in °F, and S.H. (specific heat) of water is 1 (see the previous paragraph).
Example 1
Calculate the required btus to take 40 Imp. gallons of water from 50°F to 140°F, such as would be occurring when initially filling and heating the water in a standard electric water heater.
Mass will be 40 Imperial gallons × 10 pounds/Imperial gallon = 400 pounds of water
ΔT will be (140°F − 50°F) = 90°F
S.H. (specific heat) of water = 1
Therefore, the heat quantity required for that job would be:
400 × 90 × 1 = 36,000 btus.
If the expectation is that this job needed to be done in an hour, then we would have to add 36,000 btuh to the water. If the job were to be compressed to within ½ hour, then we would have to double the heat quantity to meet that time frame, so we would have to apply 36,000 × 2 = 72,000 btuh.
Example 2
Calculate the heat load on a boiler that contains 20 Imperial gallons of water, if the water temperature has to be increased from 170°F to 190°F.
Mass = 20 Imp. Gallons × 10 lbs/Imp gal = 200 lbs
ΔT = (190 − 170) = 20°F
S.H. of water = 1
200 × 20 × 1 = 4,000 BTUs
Boilers used in residential hydronic (hot water) heating systems typically operate on a temperature differential (ΔT) of 20°F, unlike a storage-type water heater that normally operates on a ΔT of approximately 90 to 100°F. Traditional non-condensing boilers must keep the temperature of the water returning back into the boiler at a minimum of 140°F so as to keep condensation from forming on the external surfaces of the heat exchanger. Condensation is caused when the large amounts of water vapour produced by the burning of hydrocarbon gases, such as natural gas and propane, come in contact with a heat exchanger that, although considered hot, has a temperature below atmospheric dewpoint (approximately 129°F). Condensation would create many problems including corrosion, sooting, cooling of the flame, plugged heat exchangers and premature failure.
In the heating industry, the heat quantities required are predominantly expressed within a 1-hour time frame. Thus, our heat loads are always referenced as “BTUH”. We will look at the losses from a building and the heat we intend to put back into it in order to offset those losses, and we’ll specify those requirements in terms of BTUH.
Water vs Air as a Heating Medium
It has long been known that the use of water for heating buildings has many advantages over the use of air for the same purposes. This is covered within the Level 2 learning guide and should be revisited to get the most knowledge from this learning package. Aside from other factors discussed, the single most-utilized advantage is water’s ability to hold more heat than can air. One US gallon of water (the industry standard ) would contain approximately 167 btus if it were to be heated from 170°F to 190°F. (8.33 pounds × 20°FΔT × 1). By comparison, the volume of air required to hold the same amount of heat would be staggering. The specific heat of one cubic foot of air is 0.018 btu. In other words, it would take 0.018 btus to raise the temperature of one cubic foot of air by one degree Fahrenheit. If Q represents the amount of air in ft³ needed to hold 167 btus, and we were trying to increase temperature by 20°F, then we would apply the formula:
Q × 0.018 × 20 = 167
Working the formula through, we would calculate Q to be approximately 464 ft³.
What this means is that, in order to deliver a 20°F increase in temperature to a hot water heating system, we would only have to send out 1 US gallon of water into it, whereas we would have to deliver 464 ft³ of air to a forced air heating system in order to get the same result. A single ¾″ diameter pipe could easily and noiselessly achieve this. A warm air duct, having a great internal area and contributing far more noise to the building (along with other associated down-sides) could also deliver this same amount of heat. Ducts, however, are much harder to conceal within a structure without compromising the structure’s integrity. Also, “zoning”, which is the strategy of applying heat to only certain areas of a building as needed, is much easier to accomplish with water rather than with air. With zoning, the correct amount of heat is delivered to each area based upon that area’s identified heat losses. Although ducted air systems can be “zoned”, it is far more involved and expensive than when using water. Again, it has long been known that hydronic systems are preferred over air systems for heating purposes for just those reasons. The one downside to a hydronic system when compared to an air system was always the relative complexity of controls and heat transfer units needed when trying to provide cooling to a building using water. The same ductwork that heats a house in the winter can cool the house in the summer simply by the use of an outdoor condensing unit coupled to a cooling coil mounted within the supply air duct (plenum) connected to a forced warm air furnace.
Heat Transfer
As mentioned earlier, the human body transfers heat in 5 ways, which are radiation, convection, perspiration, conduction and respiration. Seeing as a building doesn’t have lungs or sweat glands, a building’s heat is lost via radiation, conduction and convection. Ever since man discovered fire, it has been known that some materials allow heat to move through them better than others. These materials are known as thermal conductors or simply conductors. Conductors such as glass, metal and concrete have their molecules bound together fairly tightly and although the robust qualities of both metal and concrete make them well suited as construction materials, those same qualities are undesirable when trying to maintain a comfortable indoor environment. Other materials, such as fiberglass and polystyrene (“styrofoam”), don’t have such tight molecular bonds, and as such won’t conduct heat through them as readily. These materials are known as insulators, and are used in cavities between structural members in buildings to reduce the movement of heat. Fiberglass batts, polystyrene board and, more recently, sprayed-in foam are the most common materials used to insulate buildings.
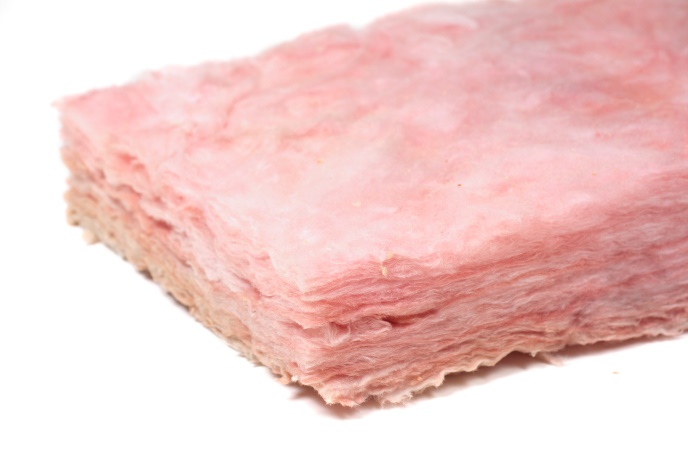
Air is also considered an insulator, as long as it can’t move freely in or out of a confined space, such as between two sealed panes of glass. For many decades now, window manufacturers have utilized the practise of creating a “dead-air space” between sealed glass panes in order to increase the insulative value of windows. Reflective coatings and the use of argon and similar inert gases increase the insulation values even further.
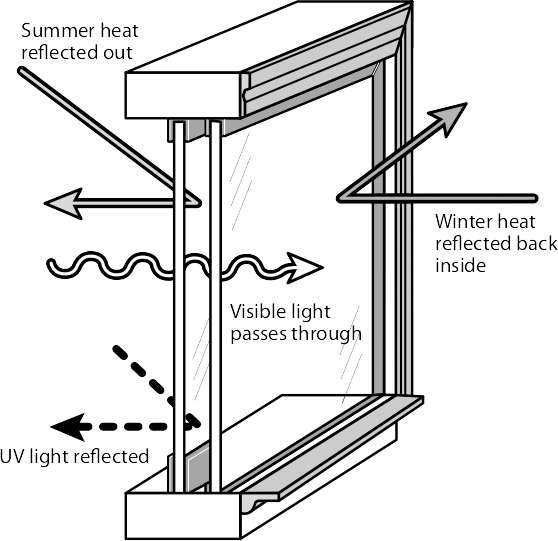
DTD (Design Temperature Difference or Differential)
The “R”, “K” and “U” values for materials are expressions of thermal resistance or conductivity for materials or assemblies when there is a 1°F differential, or “ΔT”, between the two sides or faces of that material or assembly. As mentioned earlier, if the ΔT is doubled, then the rate of heat transfer will also double. If the ΔT is halved, then so will be the rate of transfer and so on. The ΔT that is used in heat loss calculations for buildings will the temperature difference between what is desired indoors, known as “Indoor Design Temperature” or “IDT”, and the outdoor design temperature or “ODT”.
Part 9 of the BC Building Code (“BCBC”) identifies the indoor design temperature to be used in three areas of buildings.
Specifically, 9.33.3.1 (1) contains:
- subclause (a) which requires a minimum IDT of 22°C (72°F) for indoor living spaces,
- subclause (b) which requires a minimum IDT of 18°C (64°F) for unfinished basements, and
- subclause (d) which requires a minimum IDT of 15°C (59°F) for heated crawl spaces.
For ease of calculations, most designers will use 72°F for all heated areas of a residential building, however there could be cases where this may lead to the selection of a heat plant (eg. boiler) that is oversized for that application. There can be 25,000 to 50,000 btuh or more of input separating boiler models. If the heat required for the building is just above what a certain desired boiler’s output can deliver, the designer has the ability to re-evaluate or “fine tune” the heat loss in areas such as unfinished basements and heated crawlspaces, using their specific IDTs. This could possibly lower the heat loss estimate to a point which would allow a heat plant with smaller input to be selected while still delivering the minimum amount of heat needed for the application.
In addition, TECA’s Hydronics System Guidelines suggests that an IDT of 68°F (20°C) may be used in occupied areas where hydronic radiant heating is the primary source of heat. This is due to the fact that a room heated to 68°F through radiant means can offer the same level of comfort as one that is heated via convection to 72°F.
The BCBC specifies that the “Outdoor Design Temperature” (“ODT”) will be taken from Appendix 2 Climatic Design Data for Selected Locations in British Columbia. The value to be used is known as the “January 2.5% Value”.
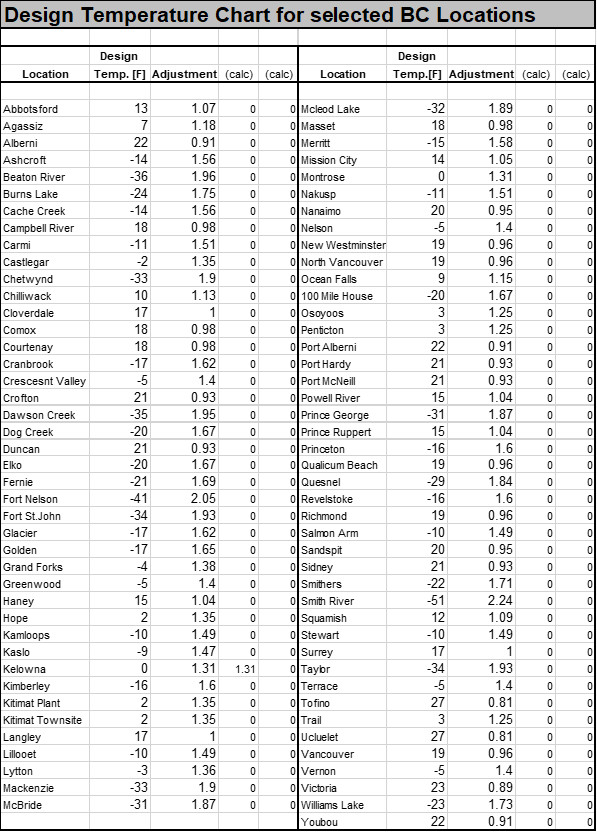
This is not the lowest recorded temperature for that location, but is the lowest expected temperature for all but 2.5%, or approximately 18 hours, of what is considered the coldest month of the year. The rationale for the 2.5% value is that buildings tend to retain their heat well enough to withstand a short period where the outdoor temperature drops below the 2.5% temperature value without dire consequences. If needed, extra layers of clothing and supplemental heat can assist building occupants in “weathering” these rare occasions.
“Design temperature difference” (DTD), or the calculated difference between the IDT and ODT, must be determined because it is used in each formula in estimating building heat loss. According to Table C-2 of the BCBC, a heat loss design estimate for a building in Penticton, BC would use 5°F (−15°C) as the ODT and 72°F (22°C) as the IDT. This means that each formula would have 67°F used as the DTD (ΔT).
Heat Gain
Buildings will gain heat in the same manner as they lose it. Some areas of the province, in particular those in the Okanagan valley, rely heavily upon air conditioning for summer comfort. In those cases, a heat gain estimation would be calculated much in the same way as for a heat loss, using the indoor design temperature and the outdoor “dry bulb” (ambient air) temperature to calculate a design temperature difference. Appendix “C” of the BCBC lists the July 2.5% dry bulb values for most locations within BC.
Media Attributions
- Figure 1 Fiberglass batt insulation by ITA is licensed under a CC BY-NC-SA licence.
- Figure 2 Modern double-pane sealed “low-E” window by ITA is licensed under a CC BY-NC-SA licence.
- Figure 3 Outdoor Design Temperature Chart is courtesy of TECA BC.