Chapter 11 Earthquakes
11.1 What is an Earthquake?
An earthquake is the shaking caused by the rupture (breaking) and subsequent displacement of rocks (one body of rock moving with respect to another) beneath Earth’s surface.
A body of rock that is under stress becomes deformed. When the rock can no longer withstand the deformation, it breaks and the two sides slide past each other. Most earthquakes take place near plate boundaries—but not necessarily right on a boundary—and not necessarily even on a pre-existing fault.
The engineering principle of elastic deformation, which can be used to understand earthquakes, is illustrated in Figure 11.1.1. The stress applied to a rock—typically because of ongoing plate movement—results in strain or deformation of the rock (Figure 11.1.1b). Because most rock is strong (unlike loose sand, for example), it can withstand a significant amount of deformation without breaking. But every rock has a deformation limit and will rupture (break) once that limit is reached. At that point, in the case of rocks within the crust, the rock breaks and there is displacement along the rupture surface (Figure 11.1.1c). The magnitude of the earthquake depends on the extent of the area that breaks (the area of the rupture surface) and the average amount of displacement (sliding).
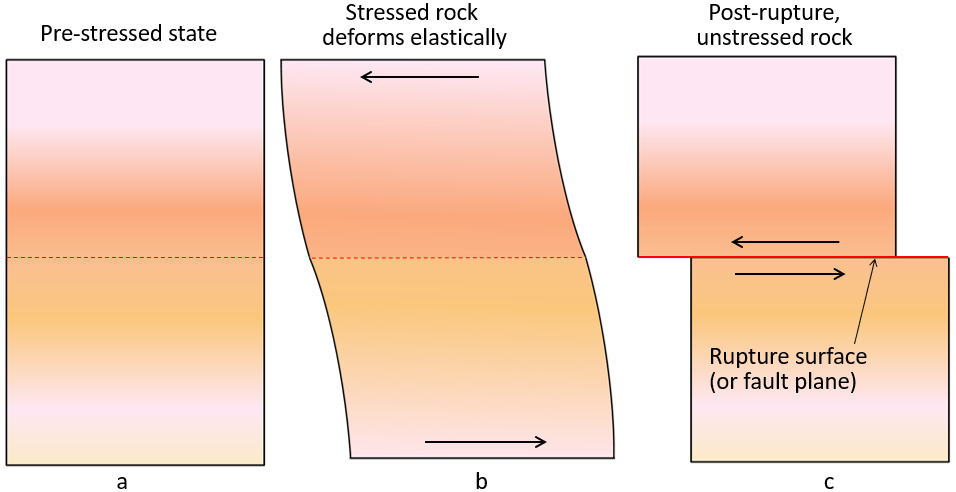
The concept of a rupture surface, which is critical to understanding earthquakes, is illustrated in Figure 11.1.2. An earthquake does not happen at a point, it happens over an area within a plane, although not necessarily a flat plane. Within the area of the rupture surface, the amount of displacement is variable (Figure 11.1.2), and, by definition, it decreases to zero at the edges of the rupture surface because the rock beyond that point isn’t displaced at all. The extent of a rupture surface and the amount of displacement will depend on a number of factors, including the type and strength of the rock, and the degree to which it was stressed beforehand.
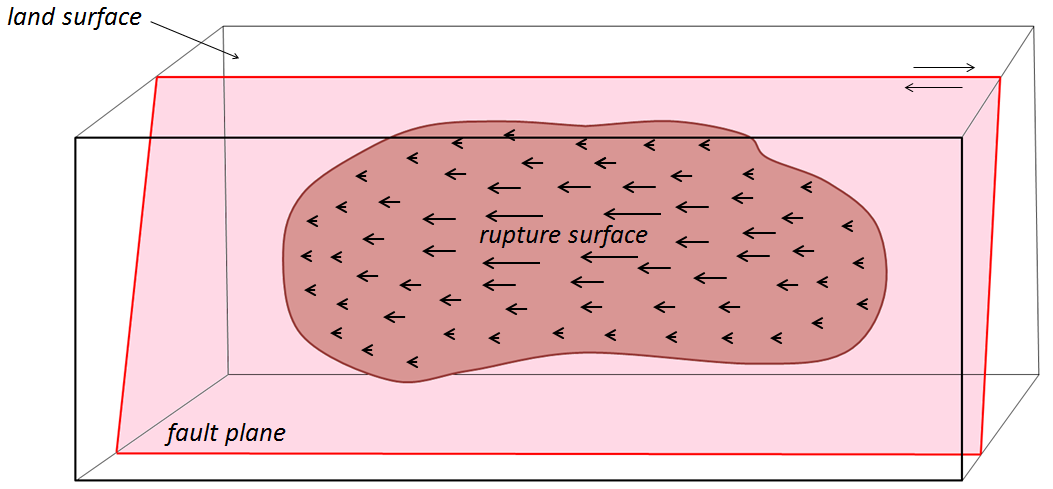
Earthquake rupture doesn’t happen all at once; it starts at a single point and spreads rapidly from there. Depending on the extent of the rupture surface, the propagation of failures out from the point of initiation is typically completed within seconds to several tens of seconds (Figure 11.1.3). The initiation point isn’t necessarily in the centre of the rupture surface; it may be close to one end, near the top, or near the bottom.
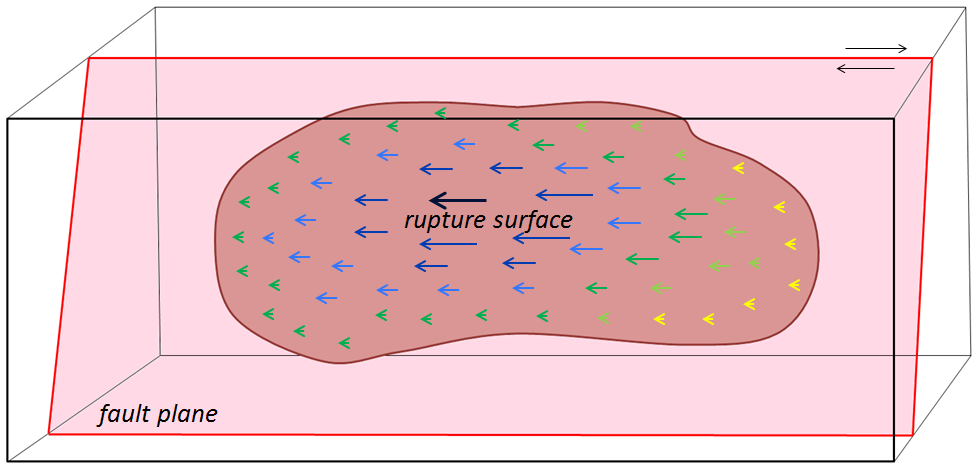
Figure 11.1.4 shows the distribution of immediate aftershocks associated with the 1989 Loma Prieta earthquake. Panel (b) is a section along the San Andreas Fault; this view is equivalent to what is shown in Figures 11.1.2 and 11.1.3. The area of red dots is the rupture surface; each red dot is a specific aftershock that was recorded on a seismometer. The hexagon labelled “main earthquake” represents the first or main shock. When that happened, the rock at that location broke and was displaced. That released the stress on that particular part of the fault, but it resulted in an increase of the stress on other nearby parts of the fault, and contributed to a cascade of smaller ruptures (aftershocks), in this case, over an area about 60 kilometres long and 15 kilometres wide.
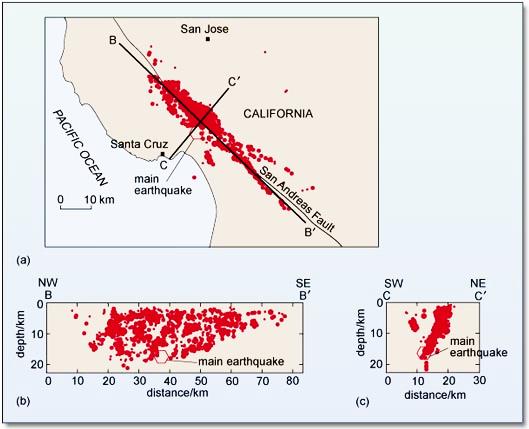
So, what exactly is an aftershock then? An aftershock is an earthquake just like any other, but it is one that can be shown to have been triggered by stress transfer from a preceding earthquake. Within a few tens of seconds of the main Loma Prieta earthquake, there were hundreds of smaller aftershocks; their distribution defines the area of the rupture surface.
Aftershocks can be of any magnitude. Most are smaller than the earthquake that triggered them, but they can be bigger. The aftershocks shown in Figure 11.1.4 all happened within seconds or minutes of the main shock, but aftershocks can be delayed for hours, days, weeks, or even years. As already noted, aftershocks are related to stress transfer. For example, the main shock of the Loma Prieta earthquake triggered aftershocks in the immediate area, which triggered more in the surrounding area, eventually extending for 30 kilometres along the fault in each direction and for 15 kilometres toward the surface. But the earthquake as a whole also changed the stress on adjacent parts of the San Andreas Fault. This effect, which has been modelled for numerous earthquakes and active faults around the world, is depicted in Figure 11.1.5. Stress was reduced in the area of the rupture (blue), but was increased at either end of the rupture surface (red and yellow).
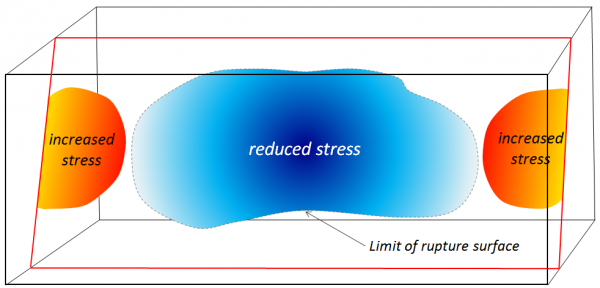
Stress transfer isn’t necessarily restricted to the fault along which an earthquake happened. It will affect the rocks in general around the site of the earthquake and may lead to increased stress on other faults in the region. The effects of stress transfer don’t necessarily show up right away. Segments of faults are typically in some state of stress, and the transfer of stress from another area is only rarely enough to push a fault segment beyond its limits to the point of rupture. The stress that is added by stress transfer accumulates along with the ongoing buildup of stress from plate motion and eventually leads to another earthquake.
Episodic Tremor and Slip
Episodic tremor and slip (ETS) is periodic slow sliding along part of a subduction boundary. It does not produce recognizable earthquakes, but does produce seismic tremor (rapid seismic vibrations on a seismometer). It was first discovered on the Vancouver Island part of the Cascadia subduction zone by Geological Survey of Canada geologists Herb Dragert and Garry Rogers.[1]
The boundary between the subducting Juan de Fuca Plate and the North America Plate can be divided into three segments (Figure 11.1.6). The cold upper part of the Juan de Fuca Plate boundary is locked. The plates are stuck and don’t move, except with very large earthquakes that happen approximately every 500 years (the last one was approximately M9 on January 26, 1700). The warm lower part of the boundary is sliding continuously because the warm rock is weaker. The central part of the boundary isn’t cold enough to be stuck, but isn’t warm enough to slide continuously. Instead it slips episodically, approximately every 14 months for about 2 weeks, moving a few centimetres each time.
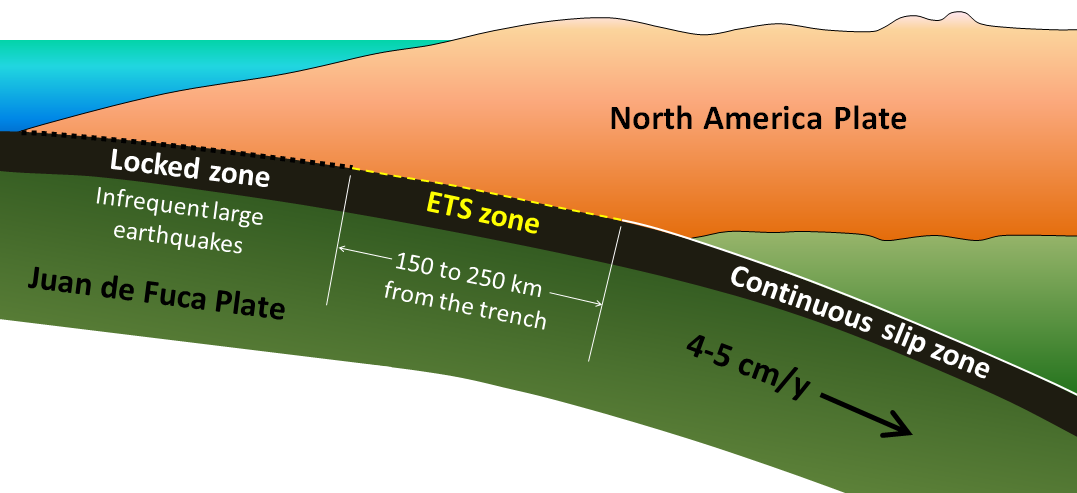
You might be inclined to think that it’s a good thing that there is periodic slip on this part of the plate because it releases some of the tension and reduces the risk of a large earthquake. In fact, the opposite is likely the case. The movement along the ETS part of the plate boundary acts like a medium-sized earthquake and leads to stress transfer to the adjacent locked part of the plate. Approximately every 14 months, during the two-week ETS period, there is a transfer of stress to the shallow locked part of the Cascadia subduction zone, and therefore an increased chance of a large earthquake.
Since 2003, ETS processes have also been observed on subduction zones in Mexico, New Zealand and Japan.
Media Attributions
- Figure 11.1.1, 11.1.2, 11.1.3, 11.1.6: © Steven Earle. CC BY.
- Figure 11.1.4: © Open University. CC BY-SA.
- Figure 11.1.5: © Steven Earle. CC BY. Based on data from 2010 Laguna Salada earthquake by Stein and Toda.
- Rogers, G. and Dragert, H., 2003, Episodic tremor and slip on the Cascadia subduction zone: the chatter of silent slip, Science, V. 300, p. 1942-1943. ↵
breaking of rock subject to stress, typically resulting in an earthquake
the deformation of material (including rock) from which it can fully recover if the stress is removed
the area over which rock rupture takes place during an earthquake
an earthquake that can be shown to have been caused by another earthquake
the change in the pattern of stress on a region of rock as a result of an earthquake (typically stress is reduced in the area of a rupture zone, but is increased elsewhere in the vicinity)