17.1 Types of Glaciers
Joyce McBeth
There are two main types of glaciers: continental glaciers and alpine glaciers. Latitude, topography, and global and regional climate patterns are important controls on the distribution and size of these glaciers.
Continental Glaciers
Continental glaciers cover vast areas of land. Today, continental glaciers are only present in extreme polar regions: Antarctica and Greenland (Figure 17.3). Historically, continental glaciers also covered large regions of Canada Europe, and Asia, and they are responsible for many distinctive topographic features in these regions (Section 17.2 and 17.3).
Continent glaciers can form and grow when climate conditions in a region cool over extended periods of time. Snow can build up over time in regions that do not warm up seasonally, and if the snow accumulates in vast amounts, it can compact under its own weight and form ice.
Earth’s two current continental glaciers, the Antarctic and Greenland Ice Sheets, comprise about 99% of Earth’s glacial ice, and approximately 68% of Earth’s fresh water. The Antarctic Ice Sheet is vastly larger than the Greenland Ice Sheet (Figure 17.4) and contains about 17 times as much ice. If the entire Antarctic Ice Sheet melted, sea level would rise by about 80 m and most of Earth’s major coastal cities would be submerged.
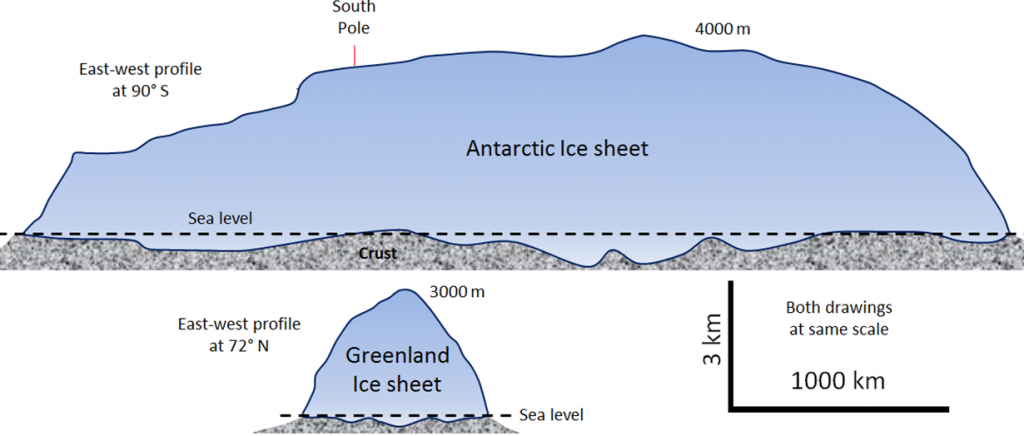
Continental glaciers generally cover areas that are flat, but the force of gravity still acts on them and causes them to flow. Continental glacier ice flows from the region where it is thickest toward the edges where it is thinner (Figure 17.5). In the central thickest parts, the ice flows almost vertically down toward the base, while at the edges of the glacier, it flows horizontally out toward the margins. In continental glaciers like the Antarctic and Greenland Ice Sheets, the thickest parts (4,000 m and 3,000 m thick, respectively) are the areas where the rate of snowfall, and therefore of ice accumulation, are greatest. In Antarctica, the ice sheet flows out over the ocean, forming ice shelves. Ice shelves can slow the flow of continental glaciers outward. Conversely, if ice shelves break down continental glacier flow can speed up.
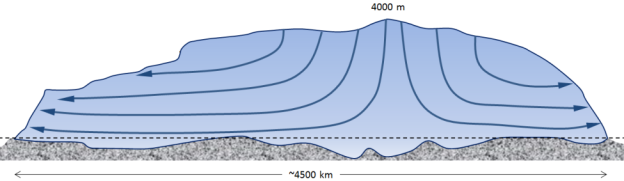
Alpine Glaciers
Alpine glaciers (also called valley glaciers) originate high up in the mountains, mostly in temperate and polar regions (Figure 17.1), but also in tropical regions in high mountains (e.g. in the Andes Mountains of South America).
The flow of alpine glaciers is driven by gravity, and primarily controlled by the slope of the ice surface (Figure 17.6). Alpine glaciers grow due to accumulation of snow over time. In the zone of accumulation, the rate of snowfall is greater than the rate of melting. In other words, not all of the snow that falls each winter melts during the following summer, and the ice surface in the zone of accumulation does not lose its annual accumulation of snow cover over the course of the year. In the zone of ablation, the rate of melting exceeds accumulation. The equilibrium line marks the boundary between the zones of accumulation (above) and ablation (below) (Figure 17.6).
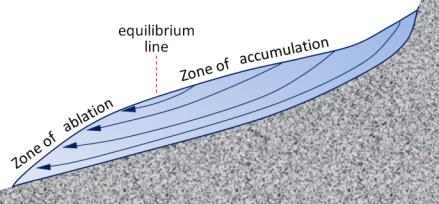
Above the equilibrium line of a glacier, winter snow will remain even after summer melting, so snow gradually accumulates on the glacier over time. The snow layer from each year is covered and compacted by subsequent snow, and it is gradually compressed and converted to firn (Figure 17.7).
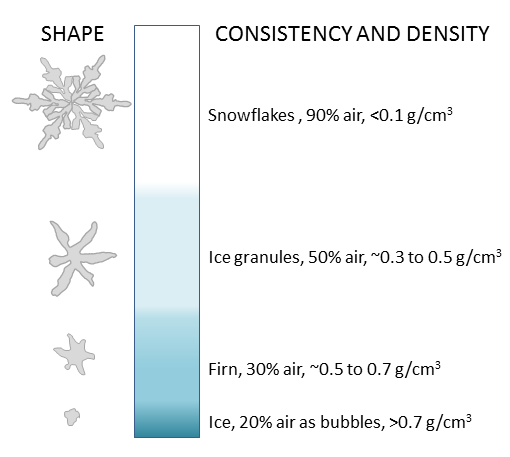
Firn is a form of ice that forms when snowflakes lose their delicate shapes and become granules due to compression. With more compression, the granules are squeezed together, and air is forced out. Eventually the granules are “welded” together to create glacial ice (Figure 17.7). Downward percolation and freezing of water from melting contributes to the process of ice formation.
The equilibrium line of a glacier near Whistler, BC, is shown in Figure 17.8. Below this line is the zone of ablation. In the zone of ablation, bare ice is exposed because the previous winter’s snow has all melted. Above this line the ice is still mostly covered with snow from the previous winter.
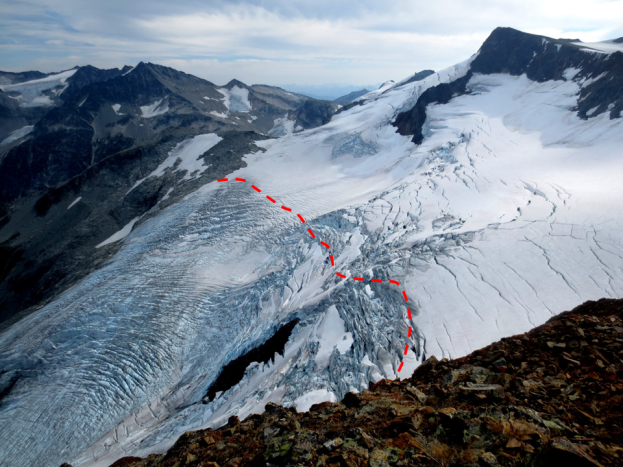
The position of the equilibrium line changes from year to year as a function of the balance between snow accumulation in the winter, and snow and ice melt during the summer. If there is more winter snow and less summer melting, this favours the advance of the equilibrium line down the glacier (and ultimately increases the size of the glacier). Between accumulation and melting, the summer melt matters most to a glacier’s ice budget. Cool summers promote an increase in glacier size, and thus lead to advance of the equilibrium line. Warm summers promote melting, and retreat of the equilibrium line.
Do You Know Your Glacier Types?
The Antarctic Ice Sheet is an example of a . These cover vast areas of land. These glaciers are “self-flattening” in the sense that gravity causes ice to flow horizontally away from thickened toward the thinner even though the glacier is on flat terrain.
You can find high in the mountains. Flow in these glaciers is controlled by the of the ice surface.
In the zone of , more snow falls each year than melts. With added pressure, snow transforms to , then , then ice. In the zone of , there’s more melting than accumulation. The marks the boundary between these zones.
Fill-in-the-blank options:
- middles
- firn
- ablation
- accumulation
- continental glacier
- slope
- granules
- equilibrium line
- alpine glaciers
- edges
To check your answers, navigate to the below link to view the interactive version of this activity.
Alpine glaciers move because they are heavy, and the force of gravity acts on the ice in the glacier to pull it down the slope of the mountains where they form. The movement of the glacier generates stress in the ice, which is proportional to the slope of the glaciers surface features of the underlying rock surface, and to the depth within the glacier.
As shown in Figure 17.9, the stresses are relatively small near the ice surface but much larger at depth. Stresses are greater in areas where the ice surface is relatively steep.
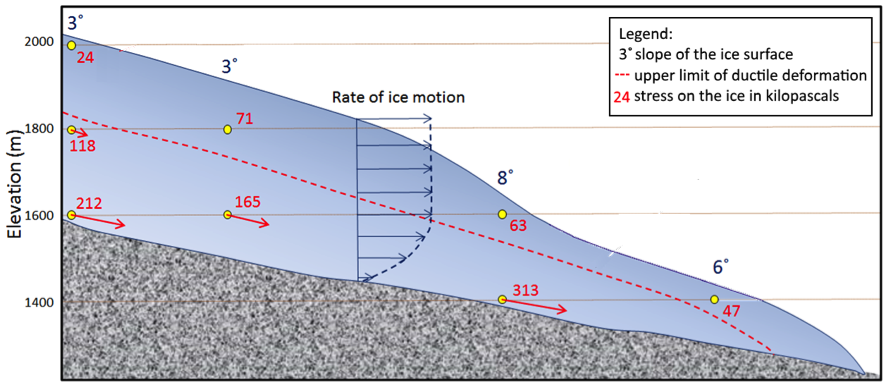
Like rock, ice behaves in a brittle fashion under low pressure conditions (shallow depths in the glacier), and plastically at higher pressures (deeper in the glacier). Stress also affects how ice deforms; at high stress ice will either break or deform plastically (ductile deformation) depending on the pressure conditions. Under brittle deformation conditions (low pressures, shallow depths in the glacier), stress is released when the ice cracks, so does not build up to high values. Within the upper 50 – 100 m of ice (above the dashed red line, in Figure 17.9), flow is brittle: the ice is rigid and will crack in response to stress. Under ductile deformation conditions (higher pressures deeper in the glacier), stress can accumulate, and the ice will flow plastically in response to that stress. Ice deforms plastically if deeper than about 100 m in the glacier, and in this region stress levels can accumulate to high values (100 kilopascals or greater, Figure 17.9).
When the lower ice of a glacier flows, it moves the upper ice along with it. It may seem from the stress patterns (red numbers and arrows in Figure 17.9) that the lower ice moves more or faster than the upper ice, but this is not the case. The lower ice deforms (flows) and the upper part is carried along and deforms through brittle deformation if subjected to sufficient stress. The upper part of the glacier moves faster than the base of the glacier because there is friction between the base of the glacier and the surface beneath it that slows the movement of the ice at the base.
The plastic lower ice of a glacier can flow over irregularities in the rocks under the glacier. However, the upper rigid ice cannot flow in this way, and because it is being carried along by the lower ice, it tends to crack in locations when the lower ice flows over changes in the topography below the glacier. This leads to formation of crevasses in areas where the rate of flow of the deeper, plastic ice is changing. In the area shown in Figure 17.10, for example, the glacier is accelerating over the steep terrain, and the rigid surface ice cracks to release stress that accumulates due to the change in velocity and tension in the ice.
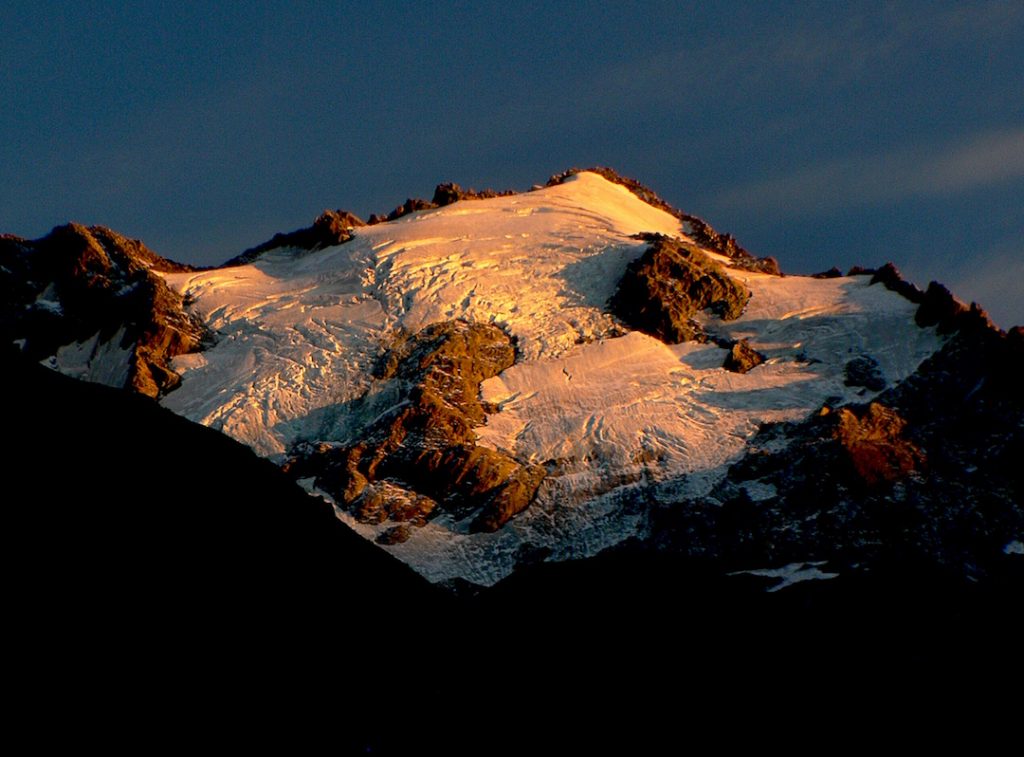
In addition to deformation, another important aspect of glacier flow is basal sliding, which is sliding movement between the base of the glacier and the underlying material. The base of a glacier can be cold (below the freezing point of water) or warm (above the freezing point). If it is warm, a film of water can form between the ice and the material underneath, and the ice will be able to slide over this surface (Figure 17.11, left). If the base is cold, the ice will be frozen to the material underneath and it will be stuck — unable to slide along its base. In this case, all the movement of the ice will be by internal flow.
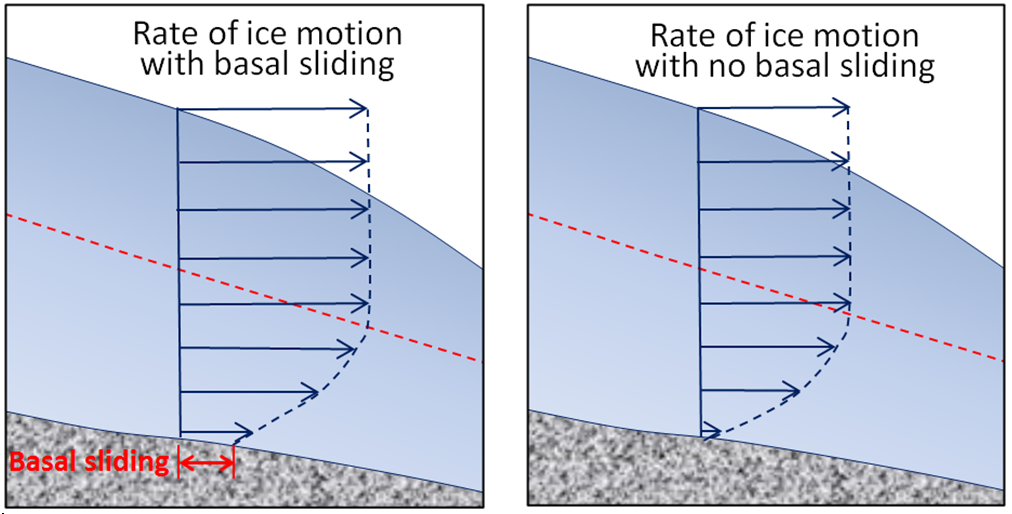
There are several factors that can influence warming of the ice and basal flow at the base of an alpine glacier. Friction between the base of the glacier and the surface underneath generates heat and can lead to melting of the ice at the base of the glacier. Rainwater and meltwater from upper regions of the glacier can percolate down and transfer heat to warm the base of the glacier and enhance basal sliding, particularly in warmer seasons. Geothermal heat from below also contributes to melting at the base of glaciers in regions with high heat flow due to volcanic activity.
Another factor that controls the temperature at the base of a glacier is the thickness of the ice. The force of gravity acting on thicker ice can enhance friction and melting at the base. Ice is also a good insulator so can prevent accumulated heat from escaping. The leading edge of an alpine glacier is typically relatively thin (see Figure 17.9), so it is common for this part to be frozen to its base while the rest of the glacier is still sliding. Since the leading edge of the glacier is frozen to the ground, and the rest of the glacier behind continues to slide forward, this causes the trailing ice to be pushed (or thrust) over top of the leading edge, forming thrust faults in the ice (Figure 17.12).
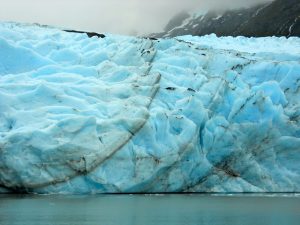
Just as the base of a glacier moves slower than the surface, the edges, which are more affected by friction along the channel walls, also move slower. If we were to place a series of markers across an alpine glacier and come back a year later, we would see that the ones in the middle had moved further forward than the ones near the edges (Figure 17.13).
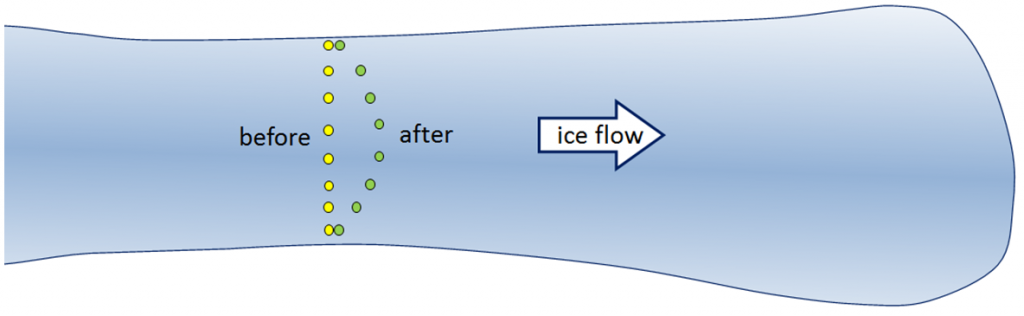
Alpine glacial ice continuously moves down the slope of the ice in response to gravity, but it may not appear to be moving because the front edge of a glacier is also continuously losing volume. It either melts or, if they glacier terminates at a lake or ocean, the front edge will calve into the water (break off pieces of the front edge of the glacier that become icebergs). If the rate of forward motion of the glacier is faster than the rate of ablation (melting), the leading edge of the glacier advances (moves forward). If the rate of forward motion is about the same as the rate of ablation, the leading edge remains stationary, and if the rate of forward motion is slower than the rate of ablation, the leading-edge retreats (moves backward).
Calving of icebergs is an important process for glaciers that terminate in lakes or oceans. An example of such a glacier is the Berg Glacier on Mt. Robson (Figure 17.14), which sheds small icebergs into Berg Lake. The Berg Glacier also lose mass by melting, evaporation, and sublimation.
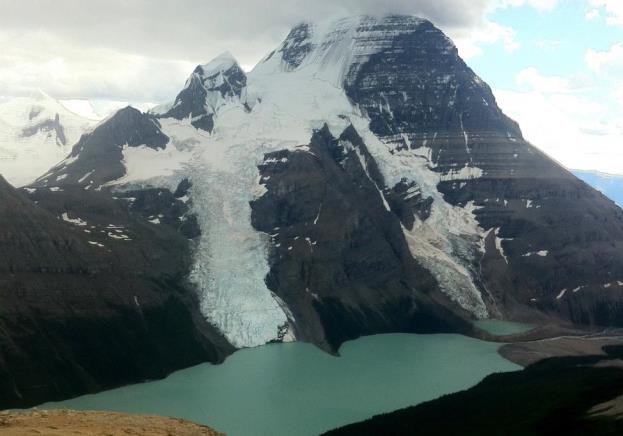
When a glacier moves, it generates stress in the ice. How the ice responds depends on how deep in the glacier it is. Within the upper 50 – 100 m, ice responds to stress with behavior, by . Deeper down, the ice deforms by instead.
If the deeper ice is flowing over irregularities in the rocks under the glacier, but the upper ice can’t flow that way, it cracks and forms as it’s carried along by the flowing ice.
Ice can move faster by if its base is above the freezing point of water, and the ice isn’t firmly attached to the rock beneath. Otherwise, it can only flow as fast as it can deform. With pressures under thick ice, this can happen even if temperatures are .
An alpine glacier that’s continuously flowing might not appear to move at all because the front edge is melting or else into water.
Fill-in-the-blank options:
- basal sliding
- crevasses
- lower
- higher
- plastically
- breaking
- brittle
- calving
- flowing
To check your answers, navigate to the below link to view the interactive version of this activity.